Population parameters and heterogeneity in survival rates of Indo-Pacific humpback dolphins in a heavily urbanized coastal region of southeast China: implications for conservation
- 1Pearl River Estuary Chinese White Dolphin National Nature Reserve, Zhuhai, China
- 2Division of Cetacean Ecology, Cetacea Research Institute, Hong Kong, Hong Kong SAR, China
- 3School of Marine Sciences, Sun Yat-Sen University, Zhuhai, China
- 4Marine Mammal and Marine Bioacoustics Laboratory, Institute of Deep-sea Science and Engineering, Chinese Academy of Sciences, Sanya, Hainan, China
- 5School of Biological Sciences, University of Hong Kong, Hong Kong, Hong Kong SAR, China
The Pearl River Delta (PRD) region on the southeast coast of China is among the largest and most populated metropolitan regions of the world, subjecting the Indo-Pacific humpback dolphins (Sousa chinensis) inhabiting coastal waters of this region – apparently the largest population of this species – to intense anthropogenic pressure. In this 5-year study (2011-2015), we applied mark-recapture techniques to quantify population parameters of humpback dolphins in the main body of the extensive estuarine system of the Pearl River, the Lingding Bay (LB), the most urbanized and industrialized part of PRD. The super-population size was estimated at 928 individuals (95% CI=823-1046), which however over-represents the latest number of extant animals. Annual estimates fluctuated between 708 and 750 dolphins, and likely reflect most accurately the latest/current number of humpback dolphins in waters of LB. Both the overall and annual estimates generated by our study are considerably lower than previously published abundance estimates. Apparent survival rates were generally low, estimated at 0.943 (SE = 0.008, 95% CI = 0.929-0.958) and 0.815 (SE = 0.025, 95% CI = 0.766-0.866) for adults and juveniles, respectively. In conjunction with limited recruitment, they may have led to a substantial decline in population numbers over the past two decades. Notably, dolphins exhibited heterogeneous apparent survival rates across the complex anthropogenic seascape of LB. Adult individuals that use Hong Kong waters as their primary habitat exhibited 6.2% higher survival rates than those in other parts of LB. This difference tripled (19.2%) for juveniles, a likely expression of their susceptibility to environmental and/or anthropogenic stresses. We suggest that the difference in survival is largely due to heterogeneous anthropogenic stressors that vary spatially across the estuary, among which habitat loss, pollution, and declining prey resources appear to be the primary threats. Based on our latest findings, we call for a strategic conservation planning with the highest priority given to preserve the remaining key dolphin habitats in Hong Kong waters, and to quantify and mitigate the impacts of major stressor(s) in Guangdong (mainland China) waters. Given the encroachment of ever-more-intense human activities on the coastal habitats of humpback dolphins, understanding their demographic response and the underlying mechanisms of the population processes may prove instrumental in the formulation of a conservation management strategy that can secure their continuous survival.
1 Introduction
Indo-Pacific humpback dolphins (Sousa chinensis, hereafter referred to as humpback dolphins) are obligatory inshore delphinids that inhabit estuarine and coastal waters off southeast Asia and western Pacific (Jefferson and Smith, 2016). With few exceptions, most extant populations of this species number only several tens or at most a few hundred of individuals (Jefferson and Smith, 2016), likely due to their dependence on shallow-water inshore habitats which are generally patchy and limited in size (Gowans et al., 2007), as seen in other species of the genus Sousa (e.g., Karczmarski et al., 2000; Parra et al., 2006). More critically, their habitats are often heavily impacted by adverse effects of various human activities, much of which concentrate in coastal regions (Lin et al., 2016; Karczmarski et al., 2017a; Karczmarski et al., 2017b; Huang et al., 2020; Lu et al., 2023). Wherever studied across the known species range, populations have been declining in numbers in recent years and decades (Reeves et al., 2008; Jefferson and Smith, 2016; Lin et al., 2022), while conservation management measures are either absent or ineffective (e.g., Karczmarski et al., 2016; Karczmarski et al., 2017a).
In waters of the Pearl River Delta (PRD) region on the southeast coast of China, humpback dolphins are found along >1000 km of coastline from Hong Kong in the east to Xiachuan Island and Yangjiang city in the west (Chen et al., 2011; Chan, 2019; Li et al., 2019) and are thought to represent the world’s largest population of this species. While human impacts on the coastal ecology of PRD go back thousands of years (Zong et al., 2009) and have been affecting humpback dolphins for centuries (Lin et al., 2016), the severity of anthropogenic impacts increased drastically since the onset of the industrial boom in mainland China in the 1990s (Zhu et al., 2013; Wu et al., 2016; Zhang et al., 2016). A greatly accelerated process of urbanization and industrialization, along with massive coastal alterations and land reclamation have degraded much of the PRD coastal habitats, especially at the eastern reaches of the region in Lingding Bay1 (Wu et al., 2016) which represents the main body of the Pearl River estuarine system. In the absence of effective environmental management strategy, major anthropogenic perturbations over the past decades have turned Lingding Bay (LB) into one of the most polluted and ecologically disturbed estuaries in the world (Li and Damen, 2010; Wang et al., 2016; Wu et al., 2016; Wang and Rainbow, 2020; Li et al., 2022). While much of the environmental health of LB has been severely compromised (Wang and Rainbow, 2020), any attempts at protection of its cetacean inhabitants have been further hampered by the lack of basic knowledge of their population demographic processes and structure (Karczmarski et al., 2016).
Much of the current knowledge of humpback dolphins in the estuarine system of the Pearl River comes from studies undertaken in late 1990s and early 2000s (e.g., Jefferson, 2000; Jefferson et al., 2006; Jefferson et al., 2012), which were conducted primarily in Hong Kong (HK) waters with only occasional surveys deeper into Lingding Bay. These early works and subsequent monitoring program in Hong Kong (e.g., Hung, 2015) along with preliminary line-transects across the PRD (Chen et al., 2010) have been used for over two decades as a primary reference to infer the status and trend of the entire population (e.g., Huang et al., 2012; Miller, 2016; Jefferson, 2018). This should be a cause for concern, given that much of the quantitatively replicable data were from Hong Kong (HK) waters that represent only a small fraction of Lingding Bay and even smaller fraction in the spatial scale of the PRD. Admittedly, in the absence of any other data, these were the only means available at the time that could facilitate any form of potential management and conservation effort. With time, however, it became increasingly evident that further studies of population processes of the PRD dolphins were much needed and at spatio-analytical scales that could provide a considerably greater data resolution than the early work could offer (Karczmarski et al., 2016). Some such studies have recently been completed and indicate a distinct socio-spatial and demographic structure of the dolphin population across the PRD (Chan, 2019; Guo et al., 2020; Chan et al., 2022a), and further fine-scale socio-spatial structure within LB where multiple clusters interact socially but remain considerably dissimilar spatially (Karczmarski et al. unpublished2). Various environmental factors also vary spatially in LB. Numerous man-made stressors differ in their type (e.g., habitat loss, pollutant load, maritime traffic, underwater noise) and intensity (e.g., extent of habitat destruction, intensity of fisheries pressure) across the anthropogenic seascape of LB (Chen et al., 2013; Wang et al., 2016; Wu et al., 2016; Yu et al., 2016; Gui et al., 2017; Pine et al., 2017; Gui et al., 2019; Guo et al., 2022; Li et al., 2022). Correspondingly, there is a considerable spatial heterogeneity in dolphin prey resources, an apparent result of heavy fishing pressure in the region (e.g., Duan et al., 2009; Lin et al., 2019; Zhou et al., 2019). Prey quantity/availability and size/quality appear to vary spatially across both PRD and LB, likely affecting the energetic costs of foraging (Zhang et al., 2019; Ho, 2021; Lin et al., 2021) which may have implications on individual fitness. Spatial heterogeneity in both resource availability and anthropogenic pressure have been attributed to spatial heterogeneity in demographic process in other taxa elsewhere (e.g., Reid et al., 2008; Harris et al., 2021; Ritterson et al., 2021). Consequently, management plans and conservation priorities in the PRD and/or LB have to be perceptively formulated with careful considerations of population structure and habitat utilization of the dolphins, as well as the quality of key dolphin habitats and levels of anthropogenic disturbance (Karczmarski et al., 2016; Karczmarski et al., 2017a). Otherwise, conservation resources and effort may be misplaced to areas of low conservation value (Freitag and Jaarsveld, 1997; Karczmarski et al., 2016).
Early studies of humpback dolphins in China applied primarily line-transect techniques to collect information such as relative abundance and distribution (e.g., Jefferson, 2000; Hung and Jefferson, 2004; Chen et al., 2008; Chen et al., 2010). Recent studies have increasingly used mark-recapture techniques for more advanced demographic analyses (e.g., Chan and Karczmarski, 2017; Chen et al., 2018; Zeng et al., 2020; Chan et al., 2022a; Lin et al., 2022; Chan and Karczmarski, 2024). Mark-recapture population modeling techniques not only deliver robust estimates of demographic parameters with considerable accuracy (e.g., Hastings et al., 2011; Pace et al., 2017; Kendall et al., 2019; Oosthuizen et al., 2019a; Schleimer et al., 2019; Bright Ross et al., 2022), but provide also powerful tools to identify the underlying driver(s) of ecological and demographic processes (e.g., Horswill et al., 2014; Oosthuizen et al., 2019b; Coxon et al., 2022; Gabriele et al., 2022; Marneweck et al., 2022; Jordaan et al., 2023) and offer a flexible framework for population monitoring that is both effective and reliable, and applicable across a wide range of taxa and management needs (e.g., Atkinson et al., 2021; Chan et al., 2022b; Goldenberg et al., 2022; Szott et al., 2022; Verborgh et al., 2022).
In the study presented here, we built a coordinated photo-identification (photo-ID) database by cross-matching multi-year data collected across Lingding Bay by research teams based in Hong Kong and mainland China. With the application of mark-recapture modelling techniques, we quantify vital population parameters of the LB humpback dolphins, and investigate the effects of age-class and individual spatial preferences on the probabilities of survival and recruitment. With the latest population size estimates delivered in this study, we construct a recent trajectory of the population by comparing previous abundance estimates (from late 1990s) with our current findings. Our study quantifies spatial variations and temporal changes of demographic parameters of humpback dolphins inhabiting LB waters, which improves our understanding of their population processes. More importantly, it provides valuable baseline information for delineating conservation management priorities in the large and complex estuarine system of Lingding Bay.
2 Materials and methods
2.1 Study area
The second largest river in China by freshwater discharge, the Pearl River, with an annual runoff of 320 billion m3 (Lu et al., 2009) enters the South China Sea through eight major distributaries in a region known as the Pearl River Delta (PRD). Four of the distributaries enter Lingding Bay (LB) and form the main body of the Pearl River estuarine system, in the east of the PRD (Figure 1). The metropolitan area that surrounds LB (including Shenzhen, Dongguan, Guangzhou, Zhongshan and Zhuhai in mainland China, and the Special Administrative Regions of Hong Kong and Macao) comprises 97.5% of the human population of the PRD economic zone, making LB one of the most urbanized and industrialized estuaries in the world (see Karczmarski et al., 2016 for more details). The present study focuses on LB, including the administrative sectors of Guangdong Province (mainland China) and Hong Kong (Figure 1). As LB represents the main body of the extensive estuarine system of the Pearl River, it is also referred to as the “Pearl River Estuary (PRE)”. It should not be mistaken, however, with what some earlier studies (e.g. Jefferson, 2000; Jefferson and Hung, 2004) referred to as PRE, as much of the earlier work was based primarily (though not exclusively) in Hong Kong waters, a small section of a much larger LB within still larger and physiographically complex PRD coastal system (e.g., Karczmarski et al., 2016; Lin et al., 2021; Chan et al., 2022a).
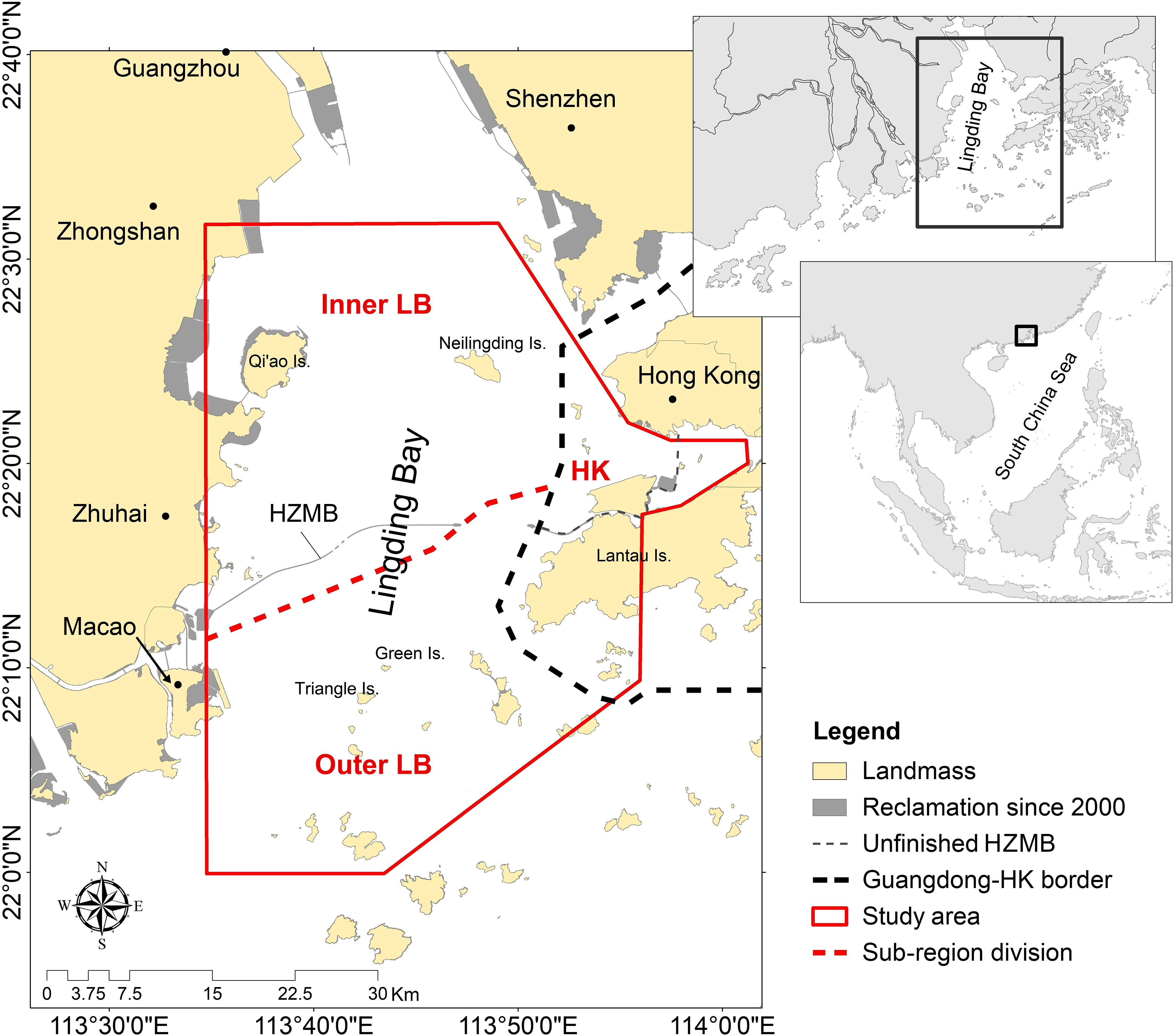
Figure 1 The study area (solid red line) in the Lingding Bay (LB), at the eastern reaches of the Pearl River Delta (PRD) region, southeast China. The thick black dashed line indicates the administrative border between Guangdong Province and Hong Kong (HK). The study area in Guangdong waters is further divided into inner Lingding Bay (iLB) and outer Lingding Bay (oLB) along the East-West shipping lane across the bay (dashed red line); see also ‘Data structure’. The Hong Kong-Zhuhai-Macao Bridge (HZMB) displayed in this figure represents the construction status at the time of this study, when the eastern section of the bridge in Hong Kong waters was not yet finished (grey dashed line).
2.2 Data collection and photographic identification
From January 2011 to December 2015, when sea conditions and weather allowed (sea state ≤ 3 in Beaufort scale and visibility ≥ 1 km), boat-based photo-identification surveys were carried out concurrently in Guangdong and Hong Kong waters, following internationally recognized protocol (e.g., Hammond et al., 1990; Karczmarski et al., 2022a; Karczmarski et al., 2022b). Once a group of dolphins was encountered, the research vessels approached the animals at speed ≤6 km/h. Photographs of dolphin dorsal fins and upper bodies were taken with at least two sets of high-speed image-stabilized digital SLR cameras (Canon EOS 1D III/IV/X) each equipped with 100-400mm f/4.5-5.6 image-stabilized zoom lens. A conscious effort was made to photo-capture both right and left sides of all group members irrespective of their distinctiveness, age-class or behavior (Chan and Karczmarski, 2017; Chan and Karczmarski, 2024). The geographic location of each encounter was recorded using global positioning system (GPS). Each group was followed for at least 10 minutes or until all group members were photographed.
To ensure equal “catchability” of all individuals, only the features on the dorsal fin and dorsal ridge that were well-visible above the water (such as the shape of dorsal fin, pattern of notches, pigmentation of the dorsal ridge and fin, and prominent injury marks) were used for individual identification (Chan and Karczmarski, 2017; Lin et al., 2018); and the image quality (Q) and individual distinctiveness (D) were rated independently (Chan et al., 2022a). The image quality of ID-photographs was rigorously assessed with a score ranging from Q1 to Q100 (adopted from Karczmarski et al., 2005) based on multiple criteria, such as how well exposed and in-focus the image was, the contrast against the background, the angle of the animal to the camera, and the proportion of dolphin body photographed above the water. The distinctiveness of individuals was rated from D0 to D5, with D0 representing individuals with no identifiable features and D5 representing highly distinctive individuals (Friday et al., 2000). A consistent application of our rigorous quality criteria along with the use of long-term reliable individually distinctive features (e.g., pigmentation of the dorsal fin and ridge, pattern of notches) and considerable survey effort (frequent surveys, whenever the weather/sea conditions allowed) facilitated an effective monitoring of any potential individual mark-change (after Karczmarski et al., 2005).
All photographs of individuals with distinctiveness D≥1 and image quality Q≥60 were matched and catalogued using the photo-ID data management program DISCOVERY (Gailey and Karczmarski, 2012; Chan et al., 2022c). To minimize any potential bias from mis-identification due to insufficient image quality and/or individual distinctiveness, only high-quality ID-photographs (Q≥70) of highly distinctive individuals (D≥3) were used for all subsequent analyses. Calves were excluded from the analytical dataset as the majority of them lack reliably recognizable features (Chan and Karczmarski, 2017; Lin et al., 2018; Guo et al., 2020; Chan and Karczmarski, 2024), but were retained in the mark ID-ratio calculation to give inference of the entire population (see section 2.6 for further details). Juveniles and adults were categorized as two age-class groups as they generally have different demographic characteristics (Currey et al., 2009; Manlik et al., 2016); see Chan and Karczmarski (2017) for detailed description/definition of calves, juveniles and adults.
2.3 Data structure
The individual sighting histories were pooled by calendar year to provide annual estimates of population parameters. While mark-recapture models assume equal demographic parameters (such as apparent survival rates and capture/recapture probabilities) for all marked individuals, this assumption is often violated in studies of marine mammals, especially in the presence of population substructure and individual heterogeneity in habitat use (Peel et al., 2016). A recent study of socio-spatial dynamics of humpback dolphins in LB (Karczmarski et al. unpublished2) identified three ‘core areas’ within the present study area (each representing a relatively un-urbanized coastal habitat), namely waters surrounding islands in the inner Lingding Bay (iLB; around Neilingding and Qi’Ao Islands) and the outer Lingding Bay (oLB; around Green and Triangle Islands), and waters off the west and southwest coast of Lantau Island in Hong Kong (HK). Although the dolphins using these three core habitats are inter-connected socially, their individual range appears restricted, with considerable long-term fidelity to their respective core areas (Karczmarski et al. unpublished2), similarly as described recently for humpback dolphins in western reaches of the PRD (Chan, 2019; Chan et al., 2022a). The variation in individual spatial preference (area/habitat use), together with the differences in the intensity of anthropogenic stressors across the region (Karczmarski et al., 2016), may lead to heterogeneity in both survival and recapture estimates, which had to be accounted for in our analytical design (see Discussion).
To examine the potential heterogeneity in population parameters, as well as the variability that might stem from the differences in survey effort, the study area was divided into three sub-regions (Figure 1): (i) Hong Kong (HK) demarcated by the HK-Guangdong administrative border, (ii) the inner LB (iLB) and (iii) outer LB (oLB), north (iLB) and south (oLB) of the East-West shipping lane between Zhuhai/Macao and Hong Kong. The East-West shipping lane demarcates also the transitional area of environmental features such as salinity (Wang and Lin, 2006), prey resource (Pine et al., 2018; Lin et al., 2019) and much of human activities (Karczmarski et al., 2016). The index of individual spatial preference of a sub-region (RPij) was calculated for all identified dolphins as follows:
where rij and ri.total represent the number of sightings of individual i in sub-region j (either HK, iLB, or oLB) and in the whole study area, respectively; while Dj and Dtotal represent the survey effort (days; Table 1) in area j and in the whole study area, respectively.
Subsequently, agglomerative hierarchical clustering (AHC) was performed based on the index of individual spatial preference RPij using four clustering methods (‘complete’, ‘average’, ‘single’, and ‘ward.D’) in the R package “hclust” (Scrucca et al., 2016). The best clustering method resulting in the highest agglomerative coefficient value was selected, and the number of clusters (K) was then identified using the silhouette algorithm by calculating the optimum of the total sum of square of agglomerative coefficient value (the squared difference between each individual from the overall mean of clusters) with K ranging from 1 to 10. Thereafter, the mark-recapture dataset was structured with individuals grouped according to the resultant clusters of spatial (sub-region) preference, which allows further examinations of the potential heterogeneity. An equilateral ternary plot was constructed to illustrate how clustered (or scattered) the dolphins are in their regional preferences. As the RP indices were corrected for the survey effort (see Equation 1), the values have to be standardized to have them displayed in the same scale on all three axes of the equilateral triangular plot. The standardized RPij indices used for the ternary plot were therefore rescaled across RPHK, RPiLB and RPoLB as follows:
2.4 Goodness-of-fit (GOF) tests
Prior to mark-recapture modelling, GOF tests were performed to estimate the variance inflation factors (, the level of overdispersion of data) of various saturated Cormack-Jolly-Seber (CJS) models (Cormack, 1964; Jolly, 1965; Seber, 1965) using the bootstrap and median- methods (Anderson et al., 1994). We started with the standard time-dependent model with the time-varying effect (‘t’) on both apparent survival rates (φ) and recapture probabilities (p), and added the age-class effect (‘Age’) and/or the individual regional preference effect (‘3site’), with the exception of age-class effect on recapture probabilities, as juveniles and adults had the same chance of being photographed under the rigorous field protocol. The value of indicates overdispersion of data while indicates acceptable fitness to the data, and the model with the lowest was used as the starting model.
2.5 Modeling apparent survival rates and recapture probabilities
Mark-recapture analyses were performed using program MARK (White and Burnham, 1999). The most parsimonious model, which shows best fit to the data, was selected based on the Akaike’s Information Criterion (AICc) or Quasi-AICc (QAICc, after the correction of ) (Burnham and Anderson, 2002). If multiple models were similarly supported (ΔQAICc< 2), weighted averages of parameters were calculated according to the AICc/QAICc weight (wi) of models (Buckland et al., 1997).
To estimate the apparent survival rates (φ) and recapture probabilities (p) of humpback dolphins, CJS models were constructed with various effects applied on both parameters. Besides the time-varying effect (‘t’), constant effect (‘.’) and age-class effect (‘Age’), regional preference effects were included as individual covariates to test the assumptions that: (1) individuals grouped in the three clusters differ in their apparent survival/recapture probabilities (‘3site’); and/or (2) dolphins seen more frequently in Guangdong waters (iLB + oLB; ‘2sitea’) or (3) in outer reaches of the LB (oLB + HK; ‘2siteb’) exhibit similar apparent survival/recapture probabilities but differ from other individuals. Survey effort effect (the total number of survey days per year; ‘effort’) was incorporated as an additional covariate for recapture probabilities.
2.6 Modeling super-population size and entry probabilities
POPAN formulation of Jolly-Seber model (Schwartz and Arnason, 1996; hereafter referred to as POPAN model) was used to estimate the super-population size (N), which represents the number of animals that used the study area as part of their range at any time during the study period, and the probability of entry (PENT), which represents the probability of an individual entering the super-population during the study period as either birth, immigration, or new identification. In the POPAN analysis, we applied the same effects on apparent survival rates and capture probabilities as in the most parsimonious CJS model, and further modeled the effects of time, regional preference and survey effort on the probabilities of entry. As GOF tests are not available for POPAN models, the value of based on that of CJS model was adopted to account for the over-dispersion of data.
Since mark-recapture analyses can only estimate the number of marked individuals (N), the total super-population size (including both marked and unmarked individuals, ) was projected by the mark ID-ratio following: , where the mark ID-ratio (the proportion of marked individuals) was estimated from a pool of 100,000 randomly selected images. The variance of was calculated following Urian et al. (2015) as given in Equation 3:
The upper and lower bounds 95% confidence intervals of were estimated with and , where C was calculated as given in Equation 4:
following Burnham et al. (1987).
3 Results
3.1 Data summary
During the 5-year study period, photo-ID data across LB were collected in 564 survey days. A total of 1287 groups of humpback dolphins were encountered, resulting with 7269 cumulative sighting records of various individuals and over 400,000 ID-images of sufficient quality (Q ≥ 60). A total of 809 highly marked (D ≥ 3) individuals were catalogued using high-quality (Q ≥ 70) images (Figure 2A), of which 107, 199 and 187 dolphins were photographed only in HK, iLB and oLB, respectively; while 236 individuals were seen in any two of the three sub-regions and 80 individuals were found in all three sub-regions across LB (Figure 2B). The mean number of sightings was 11.1 while the most frequently seen individual was photographed 73 times; and 655 individuals (81%) were photographed more than once. The rate of identifying new individuals was similar in all three sub-regions (HK, iLB and oLB regional discovery curves) and remained so throughout the study (Figure 2A). The overall cumulative number of newly identified dolphins (cumulated discovery curve) climbed sharply in the first year and continued to increase, albeit at a considerably lower rate through the end of the study period (Figure 2A).
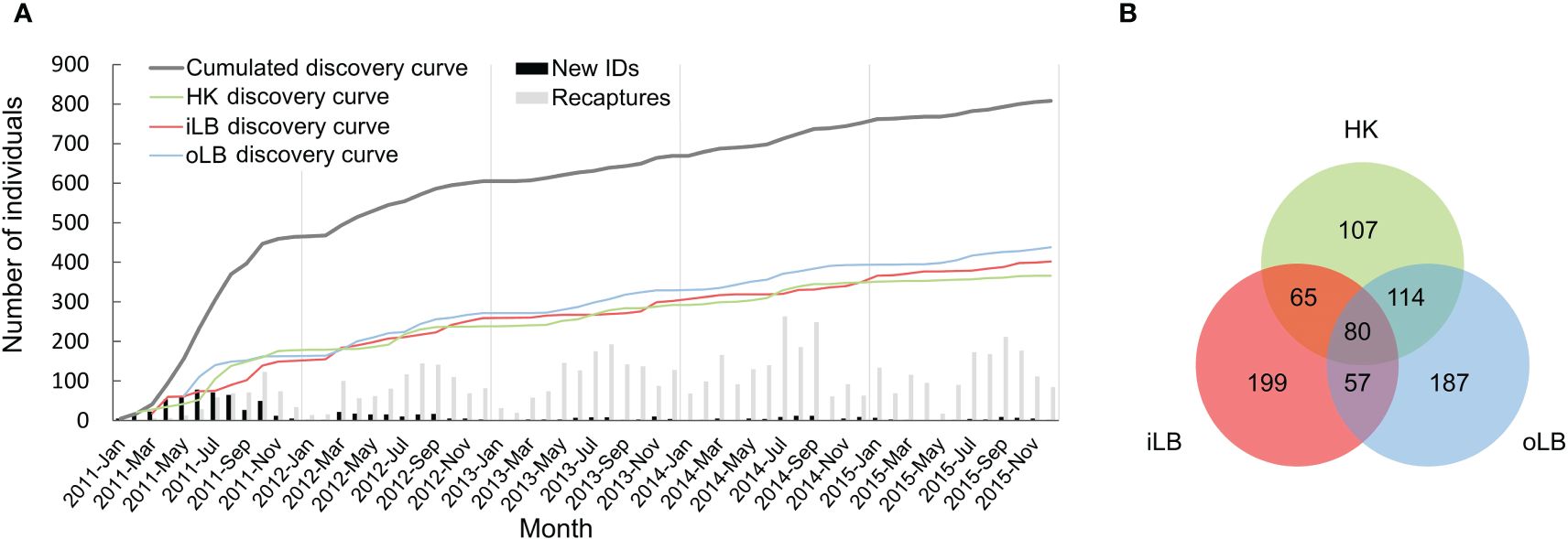
Figure 2 (A) The overall cumulative discovery curve (dark grey line) and three regional discovery curves (green, red, and blue lines) of well-marked (D ≥ 3) Indo-Pacific humpback dolphins photo-identified with high-quality (Q ≥ 70) images in Lingding Bay (LB) during five years from 2011 to 2015. The small number of newly identified dolphins (black bars) and the high number of recaptured individuals per month (light grey bars) indicate good coverage of the population in the present study. (B) Graphic summary of sightings of the 809 well-marked (D ≥ 3) humpback dolphins photo-captured in LB between 2011 and 2015, of which 107, 199 and 187 were photographed only in HK (green circle), iLB (red circle) and oLB (blue circle), respectively; while 80 individuals were seen in all three sub-regions across LB.
3.2 Agglomerative hierarchical clustering analysis
All four AHC methods estimated agglomerative coefficient close to one (‘complete’: 0.997; ‘average’: 0.994; ‘single’: 0.998; ‘ward.D’: 0.999), providing a strong indication of a spatial structure among dolphins inhabiting Lingding Bay. Using the best performing AHC method (‘ward.D’), three clusters were identified (Figure 3) corresponding to individual dolphin preferences (regional preference, RP) of specific sub-regions (Table 2). Although a small number of individuals displayed similar preference to more than one sub-region (at the center of Figure 3C), our clustering approach have structured the mark-recapture dataset sufficiently well to account for the over-dispersion of data (see further details in Section 3.3).
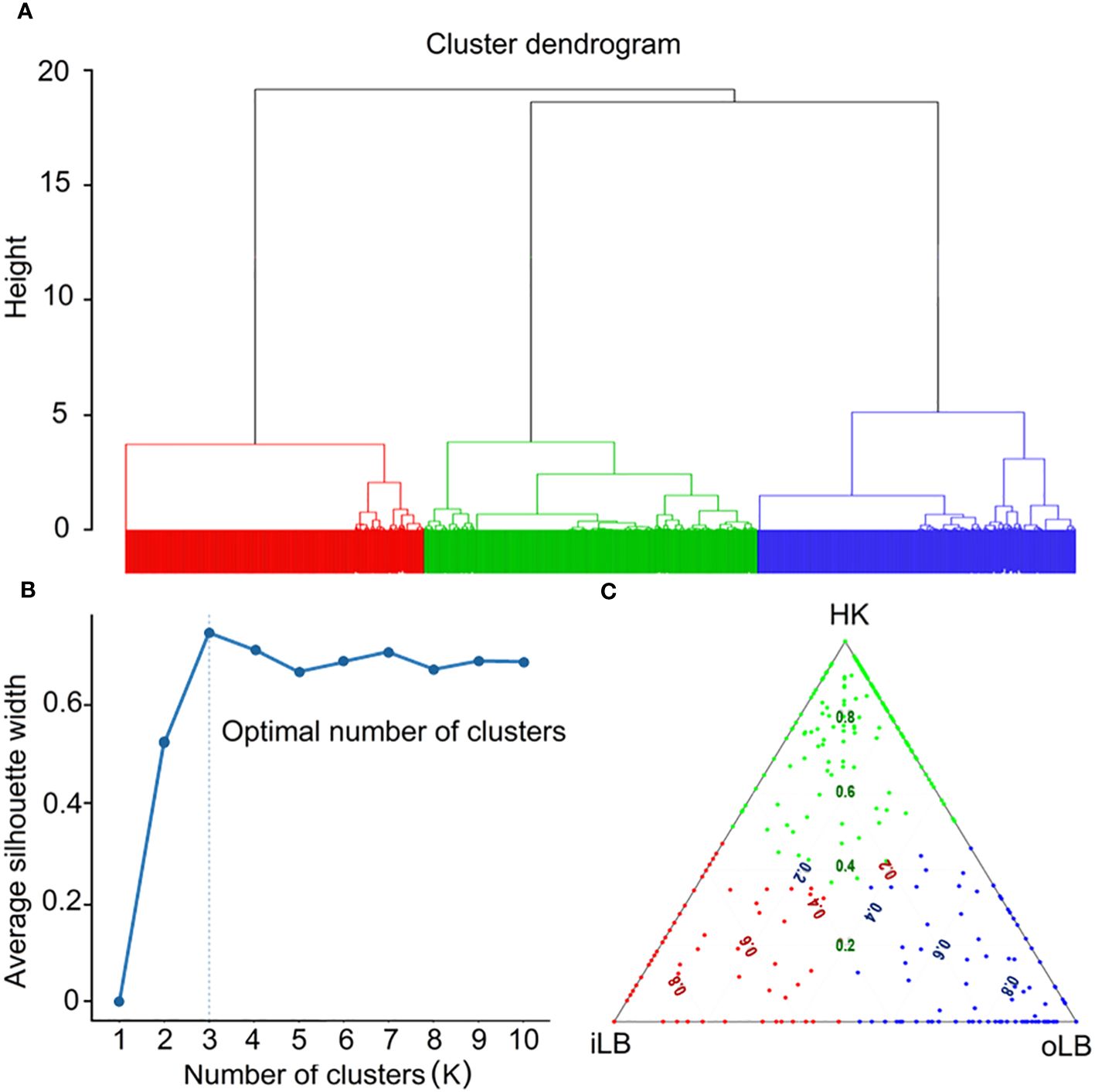
Figure 3 Agglomerative hierarchical clustering (AHC) analysis of spatial (sub-region) preferences of Indo-Pacific humpback dolphins in Lingding Bay (LB). The dendrogram (A) indicates three clusters of individuals identified using the ‘ward.D’ clustering method (the color of clusters corresponds to colors in Figures 2A, B, and panel (C)), where the optimal number of clusters (B) is determined using the silhouette algorithm. The ternary plot (C) displays the distribution of the regional preference of dolphins to the three sub-regions, inner LB (iLB), outer LB (oLB) and Hong Kong (HK), where the coloured dots indicate individuals of the three clusters. The numeric values of individual regional preference (RP) indices shown in panel (C) were standardised according to Equation 2 (see Methods section 2.3) in order to have them displayed in the same scale on all three axes of the equilateral trianglar plot.
3.3 GOF test
When only time-varying effect (‘t’) was considered, the fit of the dataset was poor with the highest level of over-dispersion of data among the models tested (Table 3). A clear asymmetry in the deviance residuals (Supplementary Figure S1) suggested that the lack of fitness was largely due to unaddressed data structure. The fit of data was improved when age-class effect (‘Age’) was incorporated to apparent survival rate parameters, and even more so when individuals were clustered into three groups according to their regional preferences (‘3site’) (; Table 3). Further improvement was only meagre when both effects of age-class and regional preference were incorporated. However, in order to include both effects to further examine the related effects in the model selection process, the model with all three effects (time-varying, age-class, and regional preference) was selected as the starting model (Table 3; see also Sections 3.4 and 3.5). To take a conservative approach, the higher value of 3.30 using the bootstrap GOF method was adopted (even though by the median- method was well below 3) to correct for QAICc during the model selection.
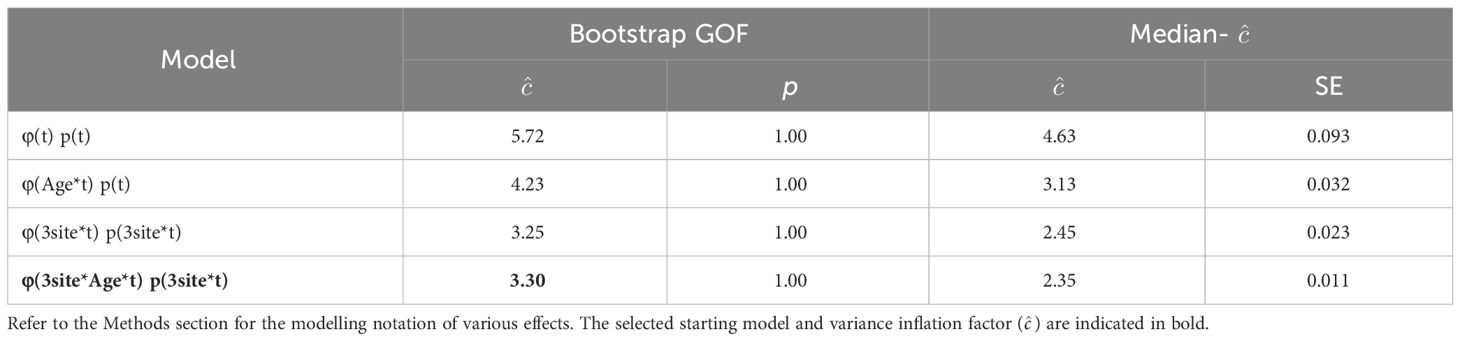
Table 3 Results of the goodness-of-fit (GOF) test of the 5-year (2011-2015) mark-recapture dataset of humpback dolphins in Lingding Bay; φ: apparent survival rate, p: recapture probability.
3.4 Apparent survival rates and recapture probabilities
The most parsimonious (best-fit) CJS candidate model presented the QAICc weight evidence ratio (w1/w2) of nearly 15 times more likely than the next model (ΔQAICc > 5, Table 4) and was therefore used for the estimation of parameters. The result of model selection suggested that the recapture probabilities varied over time (‘t’), but with little impacts of survey effort (‘effort’, model #3). Adult dolphins presented higher apparent survival rates than juveniles (‘Age’), while both survival and recapture estimates showed spatial differences (‘2sitea’, the same for iLB and oLB, but different from HK; Table 4). Dolphins that were seen more frequently in Hong Kong waters exhibited higher survival rates (HK, adults: φ = 0.980, SE = 0.005, 95% CI = 0.968-0.987; juveniles: φ = 0.926, SE = 0.018, 95% CI = 0.883-0.954) than those seen primarily in Guangdong waters (iLB and oLB, adults: φ = 0.919, SE = 0.012, 95% CI = 0.893-0.939; juveniles: φ = 0.748, SE = 0.039, 95% CI = 0.666-0.816) (Figure 4A). The difference in survival estimates between adults and juveniles was also more prominent for the iLB and oLB clusters (individuals with preference to Guangdong waters) than the HK cluster (Figure 4A). When averaged across sub-regions based on the covariate of individual regional preference, the apparent survival rates were estimated at 0.943 (SE = 0.008, 95% CI = 0.929-0.958) and 0.815 (SE = 0.025, 95% CI = 0.766-0.866) for adults and juveniles, respectively. While the overall recapture probabilities for all individuals remained high throughout the study period (p = 0.762-0.861 at all times), the estimates for the HK cluster (p = 0.844-0.915) were noticeably higher than for iLB and oLB clusters (p = 0.629-0.830). Moreover, the recapture rates in HK were relatively stable, while the estimates in iLB and oLB decreased across years (Figure 4B).
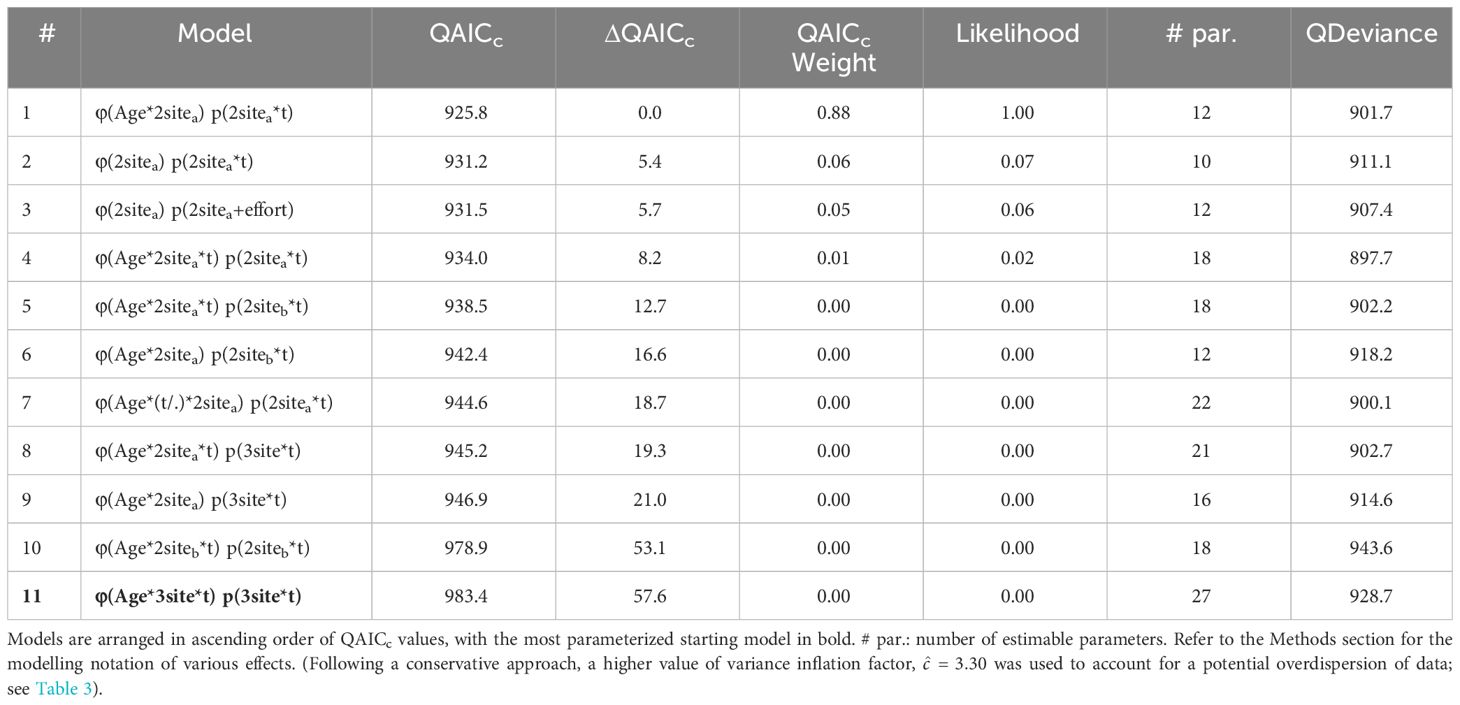
Table 4 Selection of 11 Cormack-Jolly-Seber (CJS) candidate models in estimating the apparent survival rates (φ) and recapture probabilities (p) of Indo-Pacific humpback dolphins in Lingding Bay during the period 2011-2015.
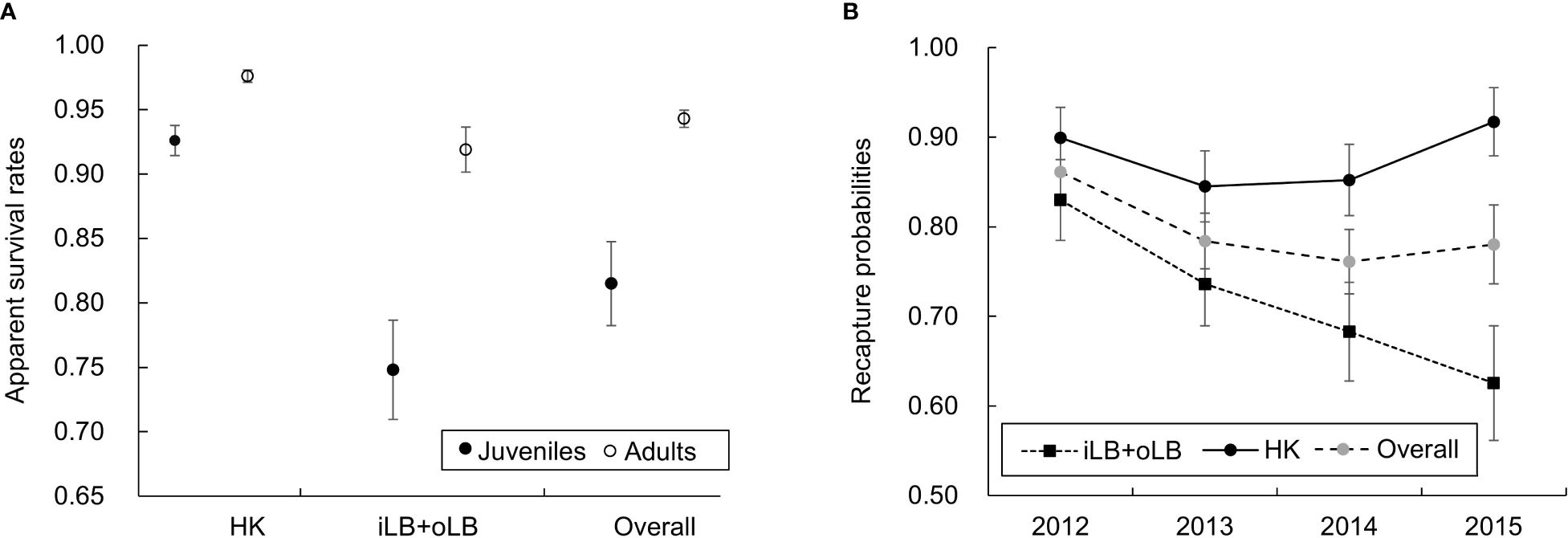
Figure 4 Estimates of (A) apparent survival rates and (B) recapture probabilities from the most parsimonious Cormack-Jolly-Seber (CJS) model. The apparent survival rates (A) show differences between age-classes (adults vs. juveniles) as well as spatially across the LB (iLB+oLB vs. HK); while the recapture rates (B) show both temporal (across years) and spatial (iLB+oLB vs. HK) differences. The overall apparent survival rates and recapture probabilities represent the averaged estimates across the sub-regions of LB. Error bars indicate standard errors (SE) of estimates.
3.5 Population size and entry probabilities
Adopting the effects on apparent survival rates and recapture probabilities of the most parsimonious CJS model (see Section 3.4 and model #1 in Table 4), the two best-fit POPAN candidate models accounted for 90% of model support, which suggested a mixture of age-class (‘Age’) and effort (‘effort’) effects in the estimation of entry probabilities (PENT) (Table 5). As both models (models #1 & #2) received comparable support (ΔQAICc< 2), model-averaging was performed for the estimates of both entry probabilities and super-population size (N). Entry probabilities for both juveniles (PENTjuvenile = 0.076-0.147) and adults (PENTadult = 0.029-0.057) decreased across years (Table 6) and were used to derive the annual entrance of marked individuals (NE), which ranged from 10 to 20 juveniles and 21-40 adults per year across the study period (Table 6). Super-population size estimates (N) suggested 142 (CV = 0.064, 95% CI = 124-160) and 713 (CV = 0.015, 95% CI = 692-734) highly marked (D ≥ 3) juveniles and adults, respectively. After correction for the mark-ID ratio (θ = 92.0%, SE = 0.27%), the total super-population size (NT) was estimated at 928 (CV = 0.061, 95% CI = 823-1046) humpback dolphins, including 154 juveniles and 774 adults. The annual super-population size estimates (after mark-ID ratio correction) fluctuated between 708 (CV = 0.127, 95% CI = 553-907) and 750 (CV = 0.077, 95% CI = 645-871) dolphins (Table 7).
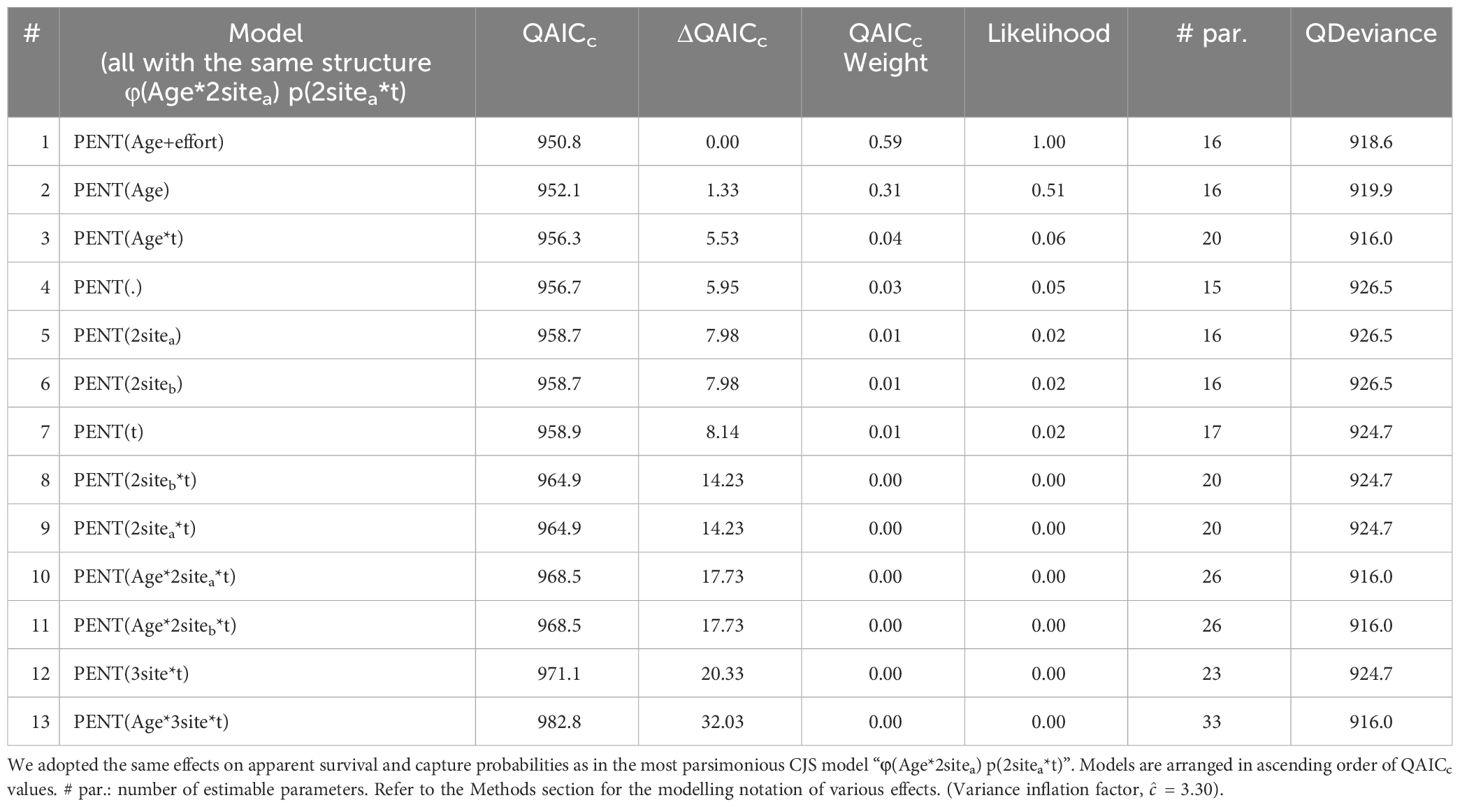
Table 5 Selection of 13 POPAN candidate models considered in estimating the apparent survival rates (φ) and capture probabilities (p), entry probabilities (PENT) and super-population size (N) of Indo-Pacific humpback dolphins in Lingding Bay during the period 2011-2015.
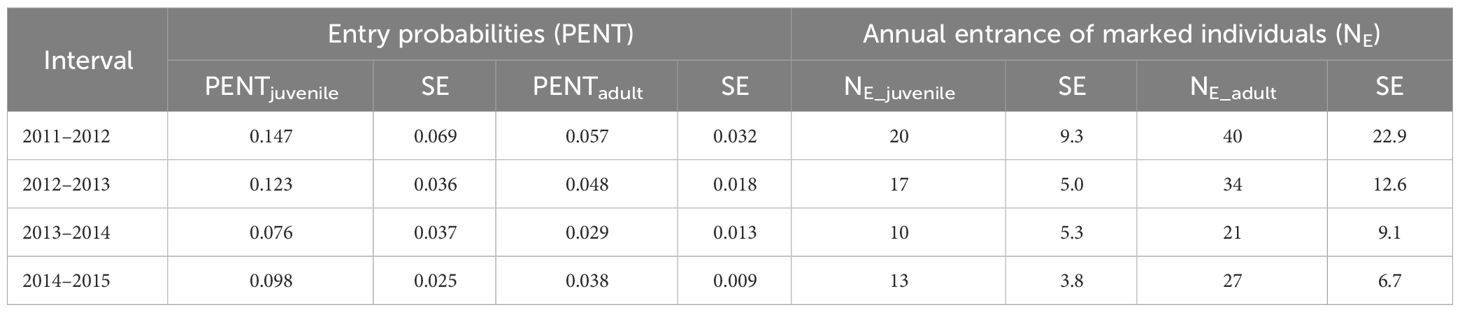
Table 6 Model-averaging estimates of entry probabilities (PENT) and numbers of annual entrance (NE) from two best-fit POPAN candidate models.
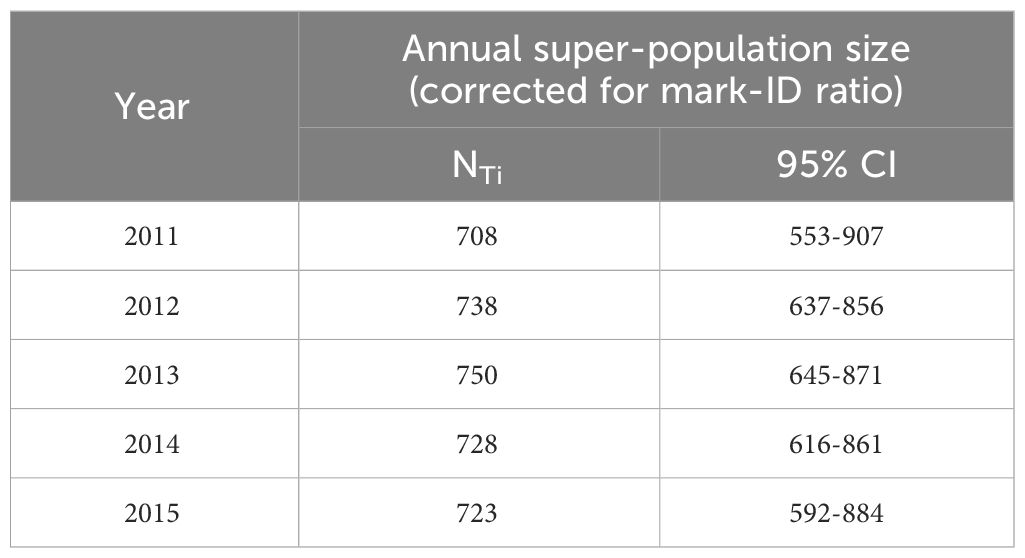
Table 7 Model-averaging estimates of annual super-population size (NTi; after correction for mark-ID ratio) from two best-fit POPAN candidate models.
4 Discussion
With the application of photo-identification mark-recapture techniques, we quantified population parameters of Indo-Pacific humpback dolphins inhabiting Lingding Bay (LB) – the main body of the Pearl River Estuary (PRE) and the most urbanized and industrialized part of the greater Pearl River Delta (PRD) region. The overall super-population size was estimated at 928 individuals, with generally low survival rates. The annual estimates – likely indicative of the latest number of humpback dolphins in LB (see further discussion) – fluctuated between 708 and 750 individuals. Both the overall and annual estimates generated by our study are considerably lower than previously published (Jefferson, 2000; Chen et al., 2010; Hung, 2015) abundance estimates (see 4.5 ‘Demographic trajectory’). The dolphins exhibited heterogeneous apparent survival rates across the complex anthropogenic seascape of LB, with the rates notably lower outside Hong Kong territorial waters.
4.1 The dataset
Heterogeneity in data structure (e.g., heterogeneity in capture probabilities), if unaccounted for, may prevent mark-recapture models from accurately fitting the sighting history data and it may introduce biases to the estimates of population parameters (Sandercock, 2006). Therefore, the robustness of models and the potential violation of the underlying assumptions needs to be carefully assessed. Given the relatively long lifespan of humpback dolphins (~38 years; Jefferson et al., 2012) as compared to the duration of our study, it was to be expected that entries of marked individuals, both adults and juveniles, declined gradually over time, dropping by half in 2014 (see Section 3.5), which corresponds to the semi-asymptotic discovery curve (Figure 2). Given the considerable population size with limited albeit continuous recruitment, the discovery curve of dolphins in LB is not expected to reach a plateau even with additional years of data. As such, the annual super-population estimates (Table 7), especially in the later years of this study that covered most individuals with a cumulated survey effort, are likely to accurately reflect the dolphin numbers in waters of LB (for comparison, see Zeng et al., 2020). Even though some LB humpback dolphins were seen moving occasionally to and from neighboring Modao Estuary, further to the west of the present study area (Chan, 2019; Chan et al., 2022a), the transition rates are exceptionally low (Chan, 2019; see also further) and potential implications on recapture probabilities, if any, can be considered negligible (Chan, 2019; Chan et al., 2022a).
We found no indication that dolphin movement may cause biases in the estimation of apparent survival rates. Firstly, movement of humpback dolphins outside LB across the PRD is limited, with less than 2% of individuals seen occasionally in waters off Macao but no further west (Chan, 2019), suggesting that downward-bias associated with permanent emigration is negligible. Secondly, dolphin movement within LB is not likely to impact estimates of survival rates, as our field surveys covered almost the entire LB across all three sub-regions. For example, prior to this study, Chan and Karczmarski (2017) modeled the demographic parameters of humpback dolphins seen in Hong Kong waters and their estimate of adult apparent survival rates (φ = 0.980) is highly consistent with the apparent survival rates of adults from the HK cluster in the present study. While the work by Chan and Karczmarski (2017) was spatially restricted to Hong Kong territorial waters only, in the present study (with a substantially larger survey area) the analytical dataset included sightings of individuals from the HK cluster in other sub-regions of LB as well, further lowering the likelihood of biases from movement and/or emigration.
Finally, recapture probabilities remained high throughout the study period and for all individuals (ranging from 0.762 to 0.861; Figure 4), minimizing the potential bias in the estimation of population parameters stemming from insufficient survey effort (Lebl et al., 2011; Lin et al., 2018).
4.2 Survival rates
Survival rates of humpback dolphins in LB are generally low, especially in individuals inhabiting Guangdong waters of LB where the survival rates are substantially lower than those estimated for other conspecific populations (Wang et al., 2012, Chen et al., 2018; Zeng et al., 2020) and several other known populations of coastal dolphins (e.g., Currey et al., 2009; Daura-Jorge et al., 2013; Lane et al., 2015). The mark-recapture survival estimates generated by our study are comparable to earlier estimates of non-calf survival rate (0.930) derived from static life-table analyses (Huang et al., 2012; Karczmarski et al., 2017a), where over 90% of samples originated from stranded carcasses recovered in LB between 1995 and 2009 (Jefferson et al., 2012). Despite the methodological differences, the continuously low survival estimates indicate that humpback dolphins inhabiting LB waters must have been under considerable stress over the past decades.
The differences in the estimated apparent survival rates of dolphins from the three sub-regions of LB are well pronounced, which is rarely reported at such a fine spatial scale (few tens of kilometers) for highly mobile animals as cetaceans. Survival rates of adults inhabiting primarily the Guangdong section of LB were estimated to be 6.2% lower than those of dolphins in HK waters, and this difference more than tripled (19.2%) for juveniles. It seems therefore apparent that humpback dolphins with spatial preference for different sub-regions of LB experience different levels of stress, either natural or anthropogenic, affecting their survival.
The individual home range of humpback dolphins (averaged at ~99.5 km2, Hung and Jefferson, 2004) comprises only a small fraction of LB estuarine waters (~2100 km2). While various anthropogenic stressors may vary in frequency and intensity across LB, the limited spatial range of individuals and their obligatory inshore distribution (Jefferson and Karczmarski, 2001; Jefferson and Smith, 2016) renders them susceptible to impacts from localized anthropogenic sources at extents corresponding to individual ranging behavior. In general, individuals living in suboptimal habitats are likely to display lower survival probabilities than those in less disturbed areas (Reid et al., 2008; Harris et al., 2021; Ritterson et al., 2021). Similarly, considerable environmental disruption, even if localized, will affect to a greater extend individuals that have used that area as part of their range compared to those whose home range lay outside the disturbed zone, and the likely implications on their survival probability may differ accordingly (Lin et al., 2023).
Since the early 1990s, the PRD economic zone has undergone rapid development and urbanization, followed by a substantial increase in human population which tripled within ten years (Ouyang et al., 2005) and continues to grow. Growing human population increases the need for food and space, which in the PRD has led to expansion of fisheries and subsequent increase in the fishery resource exploitation (Chen et al., 2013), and coastal land reclamation (especially in urban centers at the north and west of LB; Lei et al., 2002; Karczmarski et al., 2016; Zhang et al., 2016). The artificial coastline in these areas is thought to reduce fish spawning, while pollutants and suspended sediment particles from intensive dredging (which are notably higher in the Guangdong section of LB, compared to Hong Kong waters; Hong et al., 1999; Zhou et al., 2004; Chen et al., 2006; Ip et al., 2007), have weakened the fish stock recruitment by affecting the spawning rates and larval survival rates (Cai and Li, 2011). Correspondingly, in the past decade, there has been a major decline (over 70%) in fish biomass in the northernmost LB (Yu et al., 2016), the most degraded area of this estuarine ecosystem. By comparison, the coastal zone of west and southwest Lantau Island in Hong Kong underwent far less development and retains relatively natural inshore habitat (Karczmarski et al. unpublished2), representing apparently the least degraded habitat for humpback dolphins at the outer rim of LB (Karczmarski et al., 2016; Or, 2016).
The spatially-differing and locally depleted prey resources likely hamper the foraging efficiency of humpback dolphins, especially in the north and west of LB (Lin et al., 2021). This corresponds with the dolphins’ decreasing trophic level in the west of LB (Zhang et al., 2019; Ho, 2021), where individuals have to resort to feeding on much smaller prey items (Lin et al., 2021), likely undermining individual fitness. On the other hand, trophic level of humpback dolphins in Hong Kong waters appears relatively stable (Ho, 2021).
The exceptionally high level of pollutants imposes another threat to humpback dolphins in this region (Jefferson et al., 2006; Jefferson et al., 2012; Gui et al., 2014; Gui et al., 2019). Organochlorines are of particular concerns (Jefferson et al., 2006) as these endocrine disruptors are well-known for their adverse effects on reproductive and immune systems of mammals (Wells et al., 2005; Murphy et al., 2018). While it is difficult to assess the direct impacts on reproductive success across the spatial scale of the current study, high level of organochlorines likely contributes to immunosuppression which in turn may affect the dolphins’ survival, as indicated by the overall low survival rates across LB.
Intense maritime traffic in LB adds to the multitude of anthropogenic pressure on the dolphins. While a major international shipping lane lies in the east of LB (next to the Guangdong-HK administrative border), domestic ferry lanes connecting the main metropolitan centers (i.e., Hong Kong, Macau, Zhuhai, Shenzhen) crisscross the estuary. Moreover, small motor vessels that do not follow shipping lanes often cruse at high speeds in inshore waters frequented by the dolphins, causing considerable injuries that can be fatal at times (Chan and Karczmarski, 2019). The impacts of vessel collisions, behavioral disturbance and underwater noise pollution stemming from various sea traffic can be considerable (e.g., Lusseau, 2005; Bejder et al., 2006; Lusseau, 2006; Rako et al., 2013; Pine et al., 2021) and it is thought to be so for humpback dolphins in LB (Wilson et al., 2008; Jefferson and Smith, 2016; Chan and Karczmarski, 2019; Ho et al., 2024). A recent study, however, suggests that prey availability outweighs the risk posed by maritime traffic in determining the ranging behavior of LB dolphins (Pine et al., 2017). While the impact of boat traffic on dolphins’ fitness should not be underestimated, it is possible, perhaps even likely, that the pressure from other human activities, particularly habitat degradation and loss (Karczmarski et al., 2016), and depletion of prey resources in coastal waters (Lin et al., 2021), are more critical in determining the survival of the LB humpback dolphins, as reflected in the spatial variation of apparent survival rates across the estuary.
4.3 Recruitment
To minimize the potential bias of misidentification, only highly distinctive juveniles and adults were included in the analytical database. As such, new identifications resulted from either photographing individuals that have not yet been encountered before or those that were previously unmarked but gained distinctive marks over time (mostly likely juveniles). Therefore, the estimation of entry probabilities (PENT) in POPAN model considers a mixture of entries, including real immigration as well as previously non-distinctive individuals turning distinctive, without distinguishing one from the other (Arnason and Schwarz, 1999). True recruitment rates of young individuals (the combined outcome of successful birth and calf survival) are likely comparable to, if not lower than the estimated PENT for juveniles, which remained low throughout the study period (PENTjuvenile: 0.06-0.10). Given the very high mark-ID ratio (θ = 92.0%), individual identification based on multiple features (using individual-specific pattern of notches on the dorsal fin and dark spots on the upper body), and limited movements of individuals beyond the present study area (see earlier), adult immigration or mark change (change in appearance that is sufficiently large to confuse previously catalogued individual-ID) are unlikely sources of new entries.
Low recruitment rate may be ascribed to low birth rate or calf survival, or – in the case of LB humpback dolphins – more likely a combined effect of both (Jefferson et al., 2012). Most notably, the declining prey resources in LB increase the nutritional stress of humpback dolphins (Lin et al., 2021), which may consequently force the female dolphins to lower their reproductive effort as a trade-off for survival (Gunderson, 1997; Ford et al., 2010). As the fish resource deterioration in LB predates the present study for nearly a decade (see earlier section of the Discussion), the dolphins may have experienced low recruitment rates for many years (Jefferson et al., 2012), which corresponds with the aging population structure noted recently for the LB humpback dolphins (Guo et al., 2020).
Although we cannot quantitatively estimate the net birth and calf survival in the present study, the previously proposed fecundity of 0.286 (Huang et al., 2012), which is unusually high compared to other delphinid populations (0.09-0.23) (e.g. Herzing, 1997; Kogi et al., 2010; Fruet et al., 2015), is very likely overestimated given the current status of the LB dolphins. It is unlikely that a heavily impacted population of long-lived slow-reproducing species would have such high birth and calf survival rates, especially when adult survival rates – which are generally more resilient to environmental fluctuation than survival rates of young individuals (Rotella et al., 2012) – are concerningly low. Therefore, given the latest evidence presented here, earlier population risk assessments (Huang et al., 2012; Miller, 2016; Karczmarski et al., 2017a) based on the estimate of fecundity available at the time, albeit suggesting already alarmingly declining trend, were likely over-optimistic nonetheless. As aged odontocete females reproduce at lower rates (Marsh and Kasuya, 1986; Fruet et al., 2015), the LB humpback dolphins will likely continue to decline unless the majority of currently immature females become reproductive in an improved environment with considerably lower level of stress (Guo et al., 2020).
4.4 Demographic trajectory over past two decades
Since the economic “opening-up” in mainland China in 1980s, the PRD region has been transformed into a highly urbanized industrial hub of the country. The anthropogenic pressure on the estuarine system of LB has been growing ever since, and increasingly rapidly in recent decades (Chen et al., 2013; Lin et al., 2016). Demographic responses to such environmental change by its apex predator, the humpback dolphins, can be particularly revealing of long-term implications. As our mark-recapture study delivers the latest population estimates for the LB dolphins, it offers valuable insights into their current status and recent demographic trends. The overall super-population size estimate (NT = 928, 95% CI = 823-1046) represents all individuals that have used LB waters as part of their range during the time-period of this study, thus including all new entries as well as individuals that have died during that period. Given the alarmingly low survival rates (see earlier), the number of dolphins that were photographed and died during the study period is likely considerable, and thus the overall super-population size estimate over-represents the latest number of extant animals. Instead, it provides the closest inference of the initial population size during the present study period (year 2011), as it includes all identified individuals that were already in the area at the onset of the study and survived at least until encountered and photo-identified. On the other hand, the last annual super-population size estimate (NT_2015 = 723; 95% CI = 592-884) (year 2015) reflects most accurately the latest number of humpback dolphins in waters of LB, as most of new entries have likely been captured with the increasing survey effort while the individuals that have died were excluded.
While there are no other mark-recapture estimates across the entire LB area, two relative abundance estimates, both based on line-transect techniques, were available prior to this study (Jefferson, 2002; Chen et al., 2010; Hung, 2015; see Figure 5). Caution has to be taken, however, when comparing mark-recapture and line-transect estimates given the fundamental differences in the survey and analytical techniques. The estimates produced by these two techniques are only comparable when surveys, especially line-transects surveys, cover the entire range of a population (Daura-Jorge and Simões-Lopes, 2016; Lin et al., 2018), as it was the case with Chen et al. (2008) and Chen et al. (2018) estimates of humpback dolphins in Xiamen Bay. In our case, as the previous line-transect estimates covered a comparable geographic range as our current study, a comparison of the estimated number of dolphins may provide an indication of a demographic trajectory over the past two decades.
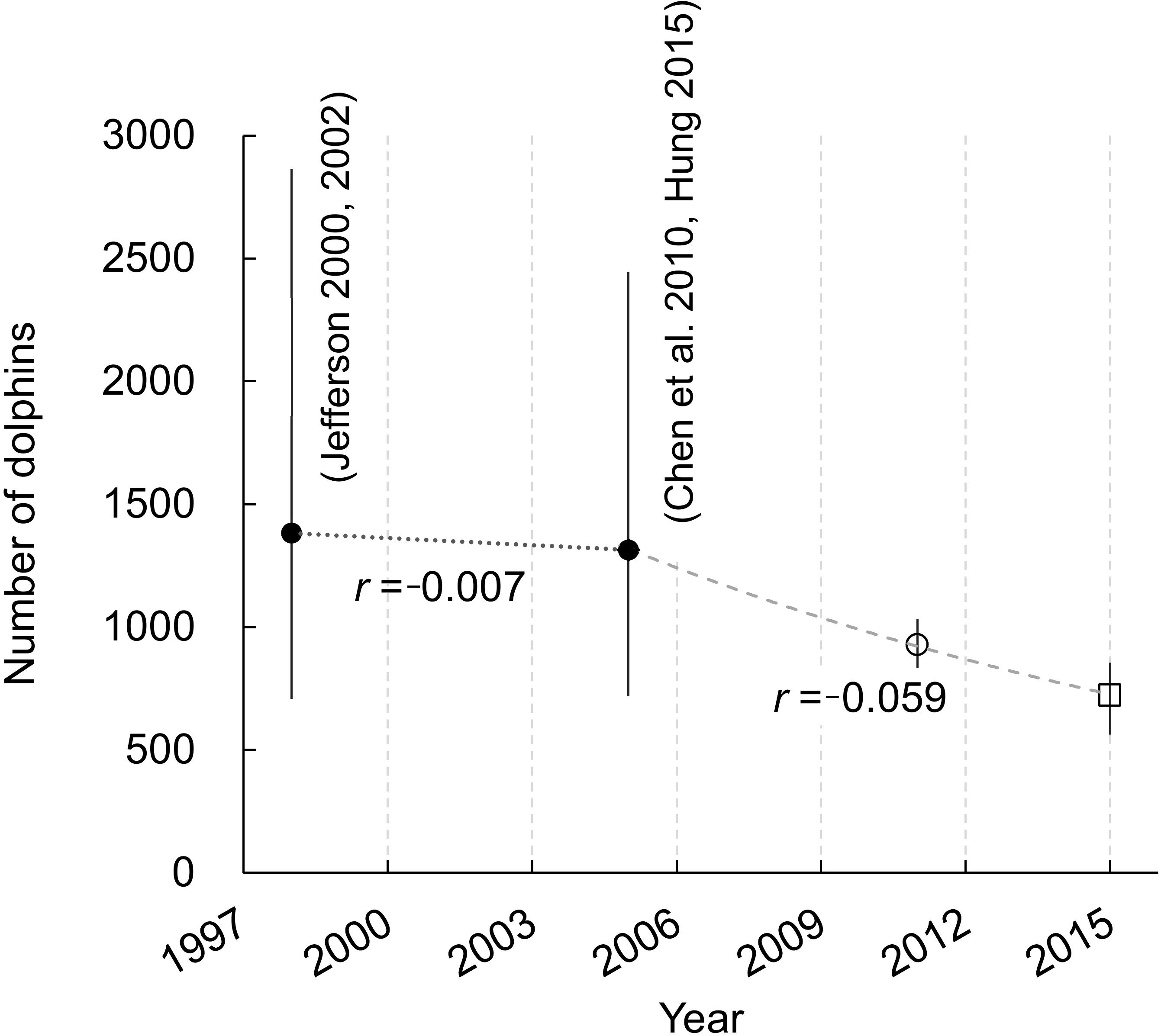
Figure 5 The inferred trajectory of Indo-Pacific humpback dolphin abundance in Lingding Bay from 1996 to 2015. The hollow circle and square indicate the super-population size estimate and the annual population size estimate of the last survey year of the present study, respectively. Black solid circles indicate two relative abundance estimates from previous line-transect studies (Jefferson, 2000; Jefferson, 2002; Chen et al., 2010; Hung, 2015). Error bars indicate the 95% confidence interval (CI) of the abundance/(super-)population size estimates. While the previous studies suffer from low precision (very large CI), if their estimates are taken for their face value, exponential regressions with least square fitting indicate accelerated rates of decline from -0.007 during 1996-2005 to -0.059 during 2005-2015.
In the 1990s, it was first estimated that there were 1382 dolphins in waters of LB (inferred from Jefferson, 2000; Jefferson, 2002), although the precision of the estimate was very low (95% CI = 708-2864). Because the data from Guangdong waters were available only for the period from November 1997 to November 1998, this estimate reflects the abundance in 1998. The second survey, which followed the exact same survey routes as Jefferson (2000), was conducted in Guangdong waters from February 2005 to January 2006 (Chen et al., 2010). Combining the abundance estimate from Chen et al. (2010) with that obtained in the same year in Hong Kong waters (Hung, 2015) gives an abundance estimate of 1314 dolphins across the LB area in 2005, albeit again with poor confidence interval (95% CI = 718-2444).
It has to be pointed out that the wide range of confidence interval of the early abundance estimates (as compared to mark-recapture estimates presented in this study; Figure 5) makes it very challenging to assess the true demographic trend. However, imperfect as they are, in the absence of any other estimates in the region, we can only infer the apparent change in dolphin numbers based on the information available. If the lack of precision of the earlier line-transect estimates were to be neglected, exponential least square fitting indicates an instantaneous rate of decline of -0.7% from 1998 to 2005, and an accelerated declining rate of -5.9% from 2005 to 2015 (Figure 5).
The magnitude of acceleration in the population decline (-0.7% vs. -5.9%) is likely exaggerated, especially as the projected rate of decline during the earlier phase based on the previous highly imprecise abundance estimates (1998-2005) was likely underestimated (Huang and Karczmarski, 2014) and lower than that reported by Huang et al. (2012) (r = -0.025). However, the accelerated declining rate in recent years is plausible, even likely, and corresponds with the ever-growing anthropogenic pressure in the PRD region, the apparently aging dolphin population (Guo et al., 2020), and low survival rates of humpback dolphins presented in this study. This puts the effectiveness of current conservation measures in LB (and across the greater PRD) in question, as discussed at length in Karczmarski et al. (2016) and Karczmarski et al. (2017a). Furthermore, the lack of confidence in projecting demographic change using the existing abundance estimates underscores the urgent need for a region-wide long-term monitoring program that could deliver accurate and precise population estimates. Only then will we be able to better understand the dolphins’ demographic response to environmental change and how it determines their long-term viability in waters of Lingding Bay.
5 Concluding comments
The inland region surrounding Lingding Bay has long been central to agriculture and industrial development throughout Chinese history (Weng, 2007; Zong et al., 2009). Currently, the urban and industrial region of the PRD accounts for at least 36% of the total trade of China (Ma and Zhang, 2009) and has become the world’s largest metropolitan area by both land area and human population size (World Bank, 2015). When faced with priority economic goals, environmental protection that is fundamental to preservation of species and habitats (Storch, 1997; Gardner et al., 2007) becomes extremely challenging (Karczmarski et al., 2017a). Recently, an ambitious development plan locally known as the ‘Greater Bay Area’ has been formulated and aims at expanding the economic development goals across the greater PRD region at unprecedented scales, which raises concerns about the fate of the region’s coastal and estuarine ecosystem in not-so-distant future.
In such an environmentally challenging situation, it is particularly important to strategize the conservation of local biodiversity with the best available science. An ongoing study (Karczmarski et al. unpublished2) has identified three major core areas of humpback dolphin habitat within LB, all of them located in inshore waters off relatively natural, uninhabited, and anthropogenically unaltered shores. Coastal waters off Lantau Island in particular comprise the largest continuous dolphin habitat in the entire LB, which matches the spatial variation in survival rates of humpback dolphins described in the present study. It is therefore of the utmost importance that all remaining dolphin core habitats in LB, majority of which are within Hong Kong waters, be given the highest conservation priority and be protected with an effective management plan as a matter of urgency. In the long-term, it is equally critical to identify and mitigate the major threats in Guangdong waters of LB, so to improve dolphin survival rates and recover the population’s healthy age structure. At present, however, in the absence of quantitative information on anthropogenic stress, it remains impractical to assess separately various sources of impacts and the dolphins’ apparent demographic response. Instead, a region-wide coordinated conservation plan is much needed to secure the ecological viability of dolphin key habitats and maintain the minimum viable number of humpback dolphins in LB waters. A continuous effort of photo-ID surveys combined with spatial analyses of habitat and resource selection is particularly important as it offers both research and management tools; it provides means to maintain vigilance of the demographic trend and data to assess the main stressors in a quantitative management-applicable manner that can inform the decision-making process of prioritizing conservation effort based on sound scientific evidence.
Data availability statement
The data used in this article may be made available by the authors upon a reasonable request.
Ethics statement
Ethical approval was not required for the study involving animals in accordance with the local legislation and institutional requirements because the present study was conducted through distant observation with no harm to the animals, and also in accordance with the local legislation and institutional requirements.
Author contributions
WL, LK and SC provided equal intellectual contribution. WL: conceptualization, data collection and management, methodology, formal analysis, data visualization, manuscript writing (initial draft), funding acquisition. LK: conceptualization and implementation of a multi-year mark-recapture study and analytical framework, data collection, validation of results, manuscript writing/revisions/edits, funding acquisition, project management, supervision of research. SC: conceptualization, data collection and management, methodology, data visualization, manuscript review/revisions/edits, funding acquisition. RZ: data collection and data processing, funding acquisition. Y-WH, YM: data collection and data processing. All authors contributed to the article and approved the submitted version.
Funding
The author(s) declare financial support was received for the research, authorship, and/or publication of this article. This work was supported by the Guangdong Natural Science Foundation grant number 2018A030313870; General Research Fund (GRF) of the Research Grants Council (RGC) of Hong Kong grant number HKU768110M and HKU17100015M; Marine Ecology Enhancement Fund (MEEF) grant number MEEF2017015, MEEF2017015A, MEEF2017015B and MEEF2017015C; Ocean Park Conservation Foundation Hong Kong (OPCFHK) grant number AW04.1617, MM01.1921, MM01.1415 and MM03.1213, the Alashan Society of Entrepreneurs and Ecology (SEE) and the Biodiversity Investigation, Observation and Assessment Program (2019-2023) of Ministry of Ecology and Environment of China (No. 9-38-27-1), and Hong Kong Airport Authority (AAHK) and Hong Kong International Airport Business Community funding CWD1_1415.
Acknowledgments
This project was partially performed during WL’s post-doctoral appointment at the University of Hong Kong. WL wishes to thank Philip Hammond for valuable suggestions at an early stage of this study; and Ruilian Zhou, Ye Lin and Ge Lin for their invaluable support.
Conflict of interest
The authors declare that the research was conducted in the absence of any commercial or financial relationships that could be construed as a potential conflict of interest.
Publisher’s note
All claims expressed in this article are solely those of the authors and do not necessarily represent those of their affiliated organizations, or those of the publisher, the editors and the reviewers. Any product that may be evaluated in this article, or claim that may be made by its manufacturer, is not guaranteed or endorsed by the publisher.
Supplementary material
The Supplementary Material for this article can be found online at: https://www.frontiersin.org/articles/10.3389/fmars.2024.1252661/full#supplementary-material
Footnotes
- ^ Lingding Bay is also referred to as the Pearl River Estuary (PRE; e.g., Jefferson 2000), or the PRE proper, as it represents the main body of the Pearl River estuarine system.
- ^ Karczmarski, L., Chan, S.C.Y., Ho, Y-W., Lin, W. (unpublished manuscript). Socio-spatial dynamics of a coastal delphinid in an anthropogenically degraded estuarine seascape.
References
Anderson D. R., Burnham K. P., White G. C. (1994). AIC model selection in overdispersed capture-recapture data. Ecology 75, 1780–1793. doi: 10.2307/1939637
Arnason A. N., Schwarz C. J. (1999). Using POPAN-5 to analyse banding data. Bird Study 46, S157–S168. doi: 10.1080/00063659909477242
Atkinson S. N., Laidre K. L., Arnold T. W., Stapleton S., Regehr E. V., Born E. W., et al. (2021). A novel mark-recapture-recovery survey using genetic sampling for polar bears Ursus maritimus in Baffin Bay. Endangered Species Res. 46, 105–120. doi: 10.3354/esr01148
Bejder L., Samuels A., Whitehead H., Gales N., Mann J., Connor R., et al. (2006). Decline in relative abundance of bottlenose dolphins exposed to long-term disturbance. Conserv. Biol. 20, 1791–1798. doi: 10.1111/j.1523-1739.2006.00540.x
Bright Ross J. G., Newman C., Buesching C. D., Macdonald D. W. (2022). Preserving identity in capture–mark–recapture studies: Increasing the accuracy of minimum number alive (MNA) estimates by incorporating inter-census trapping efficiency variation. Mamm. Biol. 102, 567–580. doi: 10.1007/s42991-021-00210-6
Buckland S. T., Burnham K. P., Augustin N. H. (1997). Model selection: an integral part of inference. Biometrics 53, 603–618. doi: 10.2307/2533961
Burnham K., Anderson D., White G., Brownie C., Pollock K. (1987). Design and analysis of fish survival experiments based on release-recapture data. Am. Fisheries Soc. Monogr. 5, 1–437.
Burnham K. P., Anderson D. R. (2002). Model selection and multimodel inference. A Practical Information-Theoretic Approach. 2nd ed (New York: Springer-Verlag).
Cai M., Li K. (2011). Economic losses from marine pollution adjacent to Pearl River Estuary, China. Proc. Eng. 18, 43–52. doi: 10.1016/j.proeng.2011.11.008
Chan S. C. Y. (2019). Demography and socio-ecology of Indo-Pacific humpback dolphin (Sousa chinensis) metapopulation in the Pearl River Estuary (Hong Kong: PhD dissertation, The University of Hong Kong).
Chan S. C. Y., Chui S. Y. S., Karczmarski L. (2022c). Application of multi−species photo−ID database management systems: A user’s perspective. Mamm. Biol. 102, 1619–1629. doi: 10.1007/s42991-023-00345-8
Chan S. C. Y., Chui S. Y. S., Pretorius Y., Karczmarski L. (2022b). Estimating population parameters of African elephants: A photographic mark-recapture application in a South African protected area. Mamm. Biol. 102, 1231–1247. doi: 10.1007/s42991-022-00334-3
Chan S. C. Y., Karczmarski L. (2017). Indo-Pacific humpback dolphins (Sousa chinensis) in Hong Kong: Modelling demographic parameters with mark-recapture techniques. PloS One 12, e0174029. doi: 10.1371/journal.pone.0174029
Chan S. C. Y., Karczmarski L. (2019). Epidermal lesions and injuries of coastal dolphins as indicators of ecological health. EcoHealth 16, 576–582. doi: 10.1007/s10393-019-01428-0
Chan S. C. Y., Karczmarski L. (2024). Broad-scale impacts of coastal mega-infrastructure project on obligatory inshore delphinids: A cautionary tale from Hong Kong. Sci. Total Environment 920, 169753. doi: 10.1016/j.scitotenv.2023.169753
Chan S. C. Y., Karczmarski L., Lin W., Zheng R., Ho Y.-W., Guo L., et al. (2022a). An unknown component of a well-known population: Socio-demographic parameters of Indo-Pacific humpback dolphins (Sousa chinensis) at the western reaches of the Pearl River Delta region. Mamm. Biol. 102, 1149–1171. doi: 10.1007/s42991-022-00335-2
Chen B., Gao H., Jefferson T. A., Lu Y., Wang L., Li S., et al. (2018). Survival rate and population size of Indo-Pacific humpback dolphins (Sousa chinensis) in Xiamen Bay, China. Mar. Mammal Sci. 34, 1018–1033. doi: 10.1111/mms.12510
Chen X., Gao H., Yao X., Chen Z., Fang H., Ye S. (2013). Ecosystem health assessment in the Pearl River Estuary of China by considering ecosystem coordination. PloS One 8, e70547. doi: 10.1371/journal.pone.0070547
Chen T., Hung S. K., Qiu Y., Jia X., Jefferson T. A. (2010). Distribution, abundance, and individual movements of Indo-Pacific humpback dolphins (Sousa chinensis) in the Pearl River Estuary, China. Mammalia 74, 117–125. doi: 10.1515/mamm.2010.024
Chen S. J., Luo X. J., Mai B. X., Sheng G. Y., Fu J. M., Zeng E. Y. (2006). Distribution and mass inventories of polycyclic aromatic hydrocarbons and organochlorine pesticides in sediments of the Pearl River Estuary and the northern South China Sea. Environ. Sci. Technol. 40, 709–714. doi: 10.1021/es052060g
Chen T., Qiu Y., Jia X., Hung S. K., Liu W. (2011). Distribution and group dynamics of Indo-Pacific humpback dolphins (Sousa chinensis) in the western Pearl River Estuary, China. Mamm. Biol. (Zeitschrift für Säugetierkunde) 76, 93–96. doi: 10.1016/j.mambio.2010.01.001
Chen B., Zheng D., Zhai F., Xu X., Sun P., Wang Q., et al. (2008). Abundance, distribution and conservation of Chinese White Dolphins (Sousa chinensis) in Xiamen, China. Mamm. Biol. 73, 156–164. doi: 10.1016/j.mambio.2006.12.002
Cormack R. M. (1964). Estimates of survival from the sighting of marked animals. Biometrika 51, 429–438. doi: 10.1093/biomet/51.3-4.429
Coxon J., Arso Civil M., Claridge D., Dunn C., Hammond P. S. (2022). Investigating local population dynamics of bottlenose dolphins in the northern Bahamas and the impact of hurricanes on survival. Mamm. Biol. 102, 1133–1148. doi: 10.1007/s42991-021-00208-0
Currey R. J. C., Dawson S. M., Slooten E., Schneider K., Lusseau D., Boisseau O. J., et al. (2009). Survival rates for a declining population of bottlenose dolphins in Doubtful Sound, New Zealand: An information theoretic approach to assessing the role of human impacts. Aquat. Conservation: Mar. Freshw. Ecosyst. 19, 658–670. doi: 10.1002/aqc.1015
Daura-Jorge F. G., Ingram S. N., Simões-Lopes P. C. (2013). Seasonal abundance and adult survival of bottlenose dolphins (Tursiops truncatus) in a community that cooperatively forages with fishermen in southern Brazil. Mar. Mammal Sci. 29, 293–311. doi: 10.1111/j.1748-7692.2012.00571.x
Daura-Jorge F. G., Simões-Lopes P. C. (2016). Mark-recapture vs. line-transect abundance estimates of a coastal dolphin population: a case study of Tursiops truncatus from Laguna, southern Brazil. Latin Am. J. Aquat. Mammals 11, 133–143. doi: 10.5597/lajam00222
Duan L. J., Li S. Y., Liu Y., Moreau J., Christensen V. (2009). Modeling changes in the coastal ecosystem of the Pearl River Estuary from 1981 to 1998. Ecol. Model. 220, 2802–2818. doi: 10.1016/j.ecolmodel.2009.07.016
Ford J. K. B., Ellis G. M., Olesiuk P. F., Balcomb K. C. (2010). Linking killer whale survival and prey abundance: food limitation in the oceans' apex predator? Biol. Lett. 6, 139–142. doi: 10.1098/rsbl.2009.0468
Freitag S., Jaarsveld A. S. V. (1997). Relative occupancy, endemism, taxonomic distinctiveness and vulnerability: Prioritizing regional conservation actions. Biodiversity Conserv. 6, 211–232. doi: 10.1023/A:1018392019594
Friday N., Smith T. D., Stevick P. T., Allen J. (2000). Measurement of photographic quality and individual distinctiveness for the photographic identification of humpback whales, Megaptera Novaeangliae. Mar. Mammal Sci. 16, 355–374. doi: 10.1111/j.1748-7692.2000.tb00930.x
Fruet P. F., Genoves R. C., Möller L. M., Botta S., Secchi E. R. (2015). Using mark-recapture and stranding data to estimate reproductive traits in female bottlenose dolphins (Tursiops truncatus) of the Southwestern Atlantic Ocean. Mar. Biol. 162, 661–673. doi: 10.1007/s00227-015-2613-0
Gabriele C. M., Amundson C. L., Neilson J. L., Straley J. M., Baker C. S., Danielson S. L. (2022). Sharp decline in humpback whale (Megaptera novaeangliae) survival and reproductive success in southeastern Alaska during and after the 2014–2016 Northeast Pacific marine heatwave. Mamm. Biol. 102, 1113–1131. doi: 10.1007/s42991-021-00187-2
Gailey G., Karczmarski L. (2012). DISCOVERY: Photo-identification data-management system for individually recognizable animals. Available at: https://www.cetacea-institute.org/discovery and https://cetaecoresearch.com/research-software-discovery.html.
Gardner T. A., Barlow J., Peres C. A. (2007). Paradox, presumption and pitfalls in conservation biology: The importance of habitat change for amphibians and reptiles. Biol. Conserv. 138, 166–179. doi: 10.1016/j.biocon.2007.04.017
Goldenberg S. Z., Chege S. M., Mwangi N., Craig I., Daballen D., Douglas-Hamilton I., et al. (2022). Social integration of translocated wildlife: A case study of rehabilitated and released elephant calves in northern Kenya. Mamm. Biol. 102, 1299–1314. doi: 10.1007/s42991-022-00285-9
Gowans S., Würsig B., Karczmarski L. (2007). The social structure and strategies of delphinids: Predictions based on an ecological framework. Adv. Mar. Biol. 53, 195–294. doi: 10.1016/S0065-2881(07)53003-8
Gui D., Yu R., He X., Tu Q., Chen L., Wu Y. (2014). Bioaccumulation and biomagnification of persistent organic pollutants in Indo-Pacific humpback dolphins (Sousa chinensis) from the Pearl River Estuary, China. Chemosphere 114, 106–113. doi: 10.1016/j.chemosphere.2014.04.028
Gui D., Yu R. Q., Karczmarski L., Ding Y., Zhang H., Sun Y., et al. (2017). Spatiotemporal trends of heavy metals in Indo-Pacific humpback dolphins (Sousa chinensis) from the western Pearl River Estuary, China. Environ. Sci. Technol. 51, 1848–1858. doi: 10.1021/acs.est.6b05566
Gui D., Zhang M., Zhang T., Zhang B., Lin W., Sun X., et al. (2019). Bioaccumulation behavior and spatiotemporal trends of per- and polyfluoroalkyl substances in Indo-Pacific humpback dolphins from the Pearl River Estuary, China. Sci. Total Environ. 658, 1029–1038. doi: 10.1016/j.scitotenv.2018.12.278
Gunderson D. R. (1997). Trade-off between reproductive effort and adult survival in oviparous and viviparous fishes. Can. J. Fisheries Aquat. Sci. 54, 990–998. doi: 10.1139/f97-019
Guo L., Lin W., Zeng C., Luo D., Wu Y. (2020). Investigating the age composition of Indo-Pacific humpback dolphins in the Pearl River Estuary based on their pigmentation pattern. Mar. Biol. 167, 50. doi: 10.1007/s00227-020-3650-x
Guo L., Luo D., Yu R. Q., Zeng C., Huang N., Wang H., et al. (2022). Habitat decline of the largest known Indo-Pacific humpback dolphin (Sousa chinensis) population in poorly protected areas associated with the hypoxic zone. Front. Mar. Sci. 9, 1048959. doi: 10.3389/fmars.2022.1048959
Hammond P. S., Mizroch S. A., Donovan G. P. (1990) Individual recognition of cetaceans: Use of photo-identification and other techniques to estimate population parameters. Reports of the International Whaling Commission, Special Issue 12. Available at: https://www.vliz.be/imisdocs/publications/253951.pdf.
Harris J. P., Smith L. M., Mcmurry S. T. (2021). Interacting gradients of selection and survival probabilities to estimate habitat quality: An example using the Gray Vireo (Vireo vicinior). Ecol. Indic. 131, 108210. doi: 10.1016/j.ecolind.2021.108210
Hastings K. K., Jemison L. A., Gelatt T. S., Laake J. L., Pendleton G. W., King J. C., et al. (2011). Cohort effects and spatial variation in age-specific survival of Steller sea lions from southeastern Alaska. Ecosphere 2, 111. doi: 10.1890/ES11-00215.1
Herzing D. L. (1997). The life history of free-ranging Atlantic spotted dolphins (Stenella frontalis): Age classes, color phases, and female reproduction. Mar. Mammal Sci. 13, 576–595. doi: 10.1111/j.1748-7692.1997.tb00085.x
Ho Y.-W. (2021). Foraging ecology of Indo-Pacific humpback dolphins in the Pearl River Estuary: A multifaceted approach (Hong Kong: PhD dissertation, The University of Hong Kong).
Ho Y.-W., Lin T.-H., Akamatsu T., Karczmarski L. (2024). Fine-scale spatial variability of marine acoustic environment corresponds with habitat utilization of Indo-Pacific humpback dolphins in Hong Kong waters. Ecol. Indic. 158, 111228. doi: 10.1016/j.ecolind.2023.111228
Hong H., Chen W., Xu L., Wang X., Zhang L. (1999). Distribution and fate of organochlorine pollutants in the Pearl River Estuary. Mar. pollut. Bull. 39, 376–382. doi: 10.1016/S0025-326X(99)00081-8
Horswill C., Matthiopoulos J., Green J. A., Meredith M. P., Forcada J., Peat H., et al. (2014). Survival in macaroni penguins and the relative importance of different drivers: Individual traits, predation pressure and environmental variability. J. Anim. Ecol. 83, 1057–1067. doi: 10.1111/1365-2656.12229
Huang S.-L., Karczmarski L. (2014). “Indo-Pacific humpback dolphin: A demographic perspective of a threatened species,” in Primates and cetaceans: Field research and conservation of complex mammalian societies. Primatology Monographs 9. Eds. Yamagiwa J., Karczmarski L. (Tokyo: Springer), 249–272. doi: 10.1007/978-4-431-54523-1_13
Huang S.-L., Karczmarski L., Chen J., Zhou R., Lin W., Zhang H., et al. (2012). Demography and population trends of the largest population of Indo-Pacific humpback dolphins. Biol. Conserv. 147, 234–242. doi: 10.1016/j.biocon.2012.01.004
Huang S.-L., Wu H., Wang X., Peng C., Wang C.-C. (2020). Beware of changes: Conservation of Indo-Pacific humpback dolphins in disturbed habitats. Aquat. Conservation: Mar. Freshw. Ecosyst. 30, 1775–1782. doi: 10.1002/aqc.3417
Hung S. K. (2015). Monitoring of marine mammals in Hong Kong waters, (2014–15): Final report (1 April 2014 to 31 March 2015) (Hong Kong: Report submitted to Hong Kong SAR Agriculture, Fisheries and Conservation Department (AFCD)), 198 pp.
Hung S. K., Jefferson T. A. (2004). Ranging patterns of Indo-Pacific humpback dolphins (Sousa chinensis) in the Pearl River estuary, Peoples Republic of China. Aquat. Mammals 30, 159–174. doi: 10.1578/AM.30.1.2004.159
Ip C. C. M., Li X. D., Zhang G., Wai O. W. H., Li Y. S. (2007). Trace metal distribution in sediments of the Pearl River Estuary and the surrounding coastal area, South China. Environ. pollut. 147, 311–323. doi: 10.1016/j.envpol.2006.06.028
Jefferson T. A. (2000). Population biology of the Indo-Pacific humpbacked dolphin in Hong Kong waters. Wildlife Monogr. 144, 1–65.
Jefferson T. A. (2002). Monitoring of Indo-Pacific humpback dolphins (Sousa chinensis) in Hong Kong waters (Final report to the Hong Kong Agriculture, Fisheries and Conservation Deparment), 49.
Jefferson T. A. (2018). Hong Kong’s Indo-Pacific humpback dolphins (Sousa chinensis): Assessing past and future anthropogenic impacts and working toward sustainability. Aquat. Mammals 44, 711–728. doi: 10.1578/AM.44.6.2018.711
Jefferson T. A., Hung S. K. (2004). A review of the status of the Indo-Pacific humpback dolphin (Sousa chinensis) in Chinese waters. Aquat. Mammals 30, 149–158. doi: 10.1578/AM.30.1.2004.149
Jefferson T. A., Hung S. K., Lam P. K. S. (2006). Strandings, mortality and morbidity of Indo-Pacific humpback dolphins in Hong Kong, with emphasis on the role of organochlorine contaminants. J. Cetacean Res. Manage. 8, 181–193. doi: 10.47536/jcrm.v8i2.714
Jefferson T. A., Hung S. K., Robertson K. M., Archer F. I. (2012). Life history of the Indo-Pacific humpback dolphin in the Pearl River Estuary, southern China. Mar. Mammal Sci. 28, 84–104. doi: 10.1111/j.1748-7692.2010.00462.x
Jefferson T. A., Karczmarski L. (2001). Sousa chinensis. Mamm. Species 655, 1–9. doi: 10.1644/1545-1410(2001)655<0001:SC>2.0.CO;2
Jefferson T. A., Smith B. D. (2016). Re-assessment of the conservation status of the Indo-Pacific humpback dolphin (Sousa chinensis) using the IUCN red list criteria. Adv. Mar. Biol. 73, 1–26. doi: 10.1016/bs.amb.2015.04.002
Jolly G. (1965). Explicit estimates from capture-recapture data with both death and immigration-stochastic models. Biometrika 52, 225–247. doi: 10.1093/biomet/52.1-2.225
Jordaan R. K., Oosthuizen W. C., Reisinger R. R., de Bruyn P. J. N. (2023). The effect of prey abundance and fisheries on the survival, reproduction, and social structure of killer whales (Orcinus orca) at subantarctic Marion Island. Ecol. Evol. 13, e10144. doi: 10.1002/ece3.10144
Karczmarski L., Chan S. C. Y., Chui S. Y. S., Cameron E. Z. (2022b). Individual identification and photographic techniques in mammalian ecological and behavioural research – Part 2: Field studies and applications. Mamm. Biol. (Special Issue) 102 (4). Available at: https://link.springer.com/journal/42991/updates/24045190. 583pp.
Karczmarski L., Chan S. C. Y., Rubenstein D. I., Chui S. Y. S., Cameron E. Z. (2022a). Individual identification and photographic techniques in mammalian ecological and behavioural research – Part 1: Methods and concepts. Mamm. Biol. (Special Issue) 102 (3). Available at: https://link.springer.com/journal/42991/updates/23466526. 502pp.
Karczmarski L., Cockcroft V. G., Mclachlan A. (2000). Habitat use and preferences of Indo-Pacific humpback dolphins Sousa chinensis in Algoa Bay, South Africa. Mar. Mammal Sci. 16, 65–79. doi: 10.1111/j.1748-7692.2000.tb00904.x
Karczmarski L., Huang S.-L., Chan S. C. Y. (2017a). Threshold of long-term survival of a coastal delphinid in anthropogenically degraded environment: Indo-Pacific humpback dolphins in Pearl River Delta. Sci. Rep. 7, 42900. doi: 10.1038/srep42900
Karczmarski L., Huang S. L., Or C. K. M., Gui D., Chan S. C. Y., Lin W., et al. (2016). Humpback dolphins in Hong Kong and the Pearl River Delta: status, threats and conservation challenges. Adv. Mar. Biol. 73, 27–64. doi: 10.1016/bs.amb.2015.09.003
Karczmarski L., Huang S.-L., Wong W.-H., Chang W.-L., Chan S. C., Keith M. (2017b). Distribution of a coastal delphinid under the impact of long-term habitat loss: Indo-Pacific humpback dolphins off Taiwan’s west coast. Estuaries Coasts 2, 594–603. doi: 10.1007/s12237-016-0146-5
Karczmarski L., Würsig B., Gailey G., Larson K. W., Vanderlip C. (2005). Spinner dolphins in a remote Hawaiian atoll: social grouping and population structure. Behav. Ecol. 16, 675–685. doi: 10.1093/beheco/ari028
Kendall W. L., Stapleton S., White G. C., Richardson J. I., Pearson K. N., Mason P. (2019). A multistate open robust design: Population dynamics, reproductive effort, and phenology of sea turtles from tagging data. Ecol. Monogr. 89, e01329. doi: 10.1002/ecm.1329
Kogi K., Hishii T., Imamura A., Iwatani T., Dudzinski K. M. (2010). Demogrpahic parameters of Indo-Pacific bottlenose dolphins (Tursiops aduncus) around Mikura Island, Japan. Mar. Mammal Sci. 20, 510–526. doi: 10.1111/j.1748-7692.2004.tb01176.x
Lane S. M., Smith C. R., Mitchell J., Balmer B. C., Barry K. P., McDonald T., et al. (2015). Reproductive outcome and survival of common bottlenose dolphins sampled in Barataria Bay, Louisiana, USA, following the Deepwater Horizon oil spill. Proc. R. Soc. B: Biol. Sci. 282, 20151944. doi: 10.1098/rspb.2015.1944
Lebl K., Bieber C., Adamík P., Fietz J., Morris P., Pilastro A., et al. (2011). Survival rates in a small hibernator, the edible dormouse: a comparison across Europe. Ecography 34, 683–692. doi: 10.1111/j.1600-0587.2010.06691.x
Lei Y., Wu C., Chen J. (2002). “Reclamation and regulation in the pearl river delta, China,” in Engineered Coasts. Eds. Chen J., Eisma D., Hotta K., Walker H. J. (Dordrecht: Springer Netherlands), 199–228.
Li X., Damen M. C. J. (2010). Coastline change detection with satellite remote sensing for environmental management of the Pearl River Estuary, China. J. Mar. Syst. 82, 54–61. doi: 10.1016/j.jmarsys.2010.02.005
Li M., Wang X., Hung S. K., Xu Y., Chen T. (2019). Indo-Pacific humpback dolphins (Sousa chinensis) in the Moyang River Estuary: The western part of the world's largest population of humpback dolphins. Aquat. Conservation: Mar. Freshw. Ecosyst. 29, 798–808. doi: 10.1002/aqc.3055
Li X., Yan H., Yang Y., Xu Z., Yang. H. (2022). Spatiotemporal coastline variations in the Pearl River Estuary and the relationship with multiple human disturbances. Front. Mar. Sci. 9, 1032105. doi: 10.3389/fmars.2022.1032105
Lin W., Chan S. C. Y., Zeng C., Karczmarski L., Wu Y. (2018). Mark-recapture technique for demographic studies of Chinese white dolphins - applications and suggestions. Acta Theriologica Sin. 38, 586–596. doi: 10.16829/j.slxb.150171
Lin W., Karczmarski L., Li J., Chan S. C. Y., Guo L., Wu Y. (2019). Differential population dynamics of a coastal porpoise correspond to the fishing effort in a large estuarine system. Aquat. Conservation: Mar. Freshw. Ecosyst. 29, 223–234. doi: 10.1002/aqc.2998
Lin W., Karczmarski L., Xia J., Zhang X., Yu X., Wu Y. (2016). Increased human occupation and agricultural development accelerates the population contraction of an estuarine delphinid. Sci. Rep. 6, 35713. doi: 10.1038/srep35713
Lin W., Karczmarski L., Zhou R., Mo Y., Guo L., Yiu S. K. F., et al. (2021). Prey decline leads to diet shift in the largest population of Indo-Pacific humpback dolphins? Integr. Zoology 16, 548–574. doi: 10.1111/1749-4877.12548
Lin W., Zheng R., Liu B., Chen S., Lin M., Liu M., et al. (2022). Low survivals and rapid demographic decline of a threatened estuarine delphinid. Front. Mar. Sci. 9, 782680. doi: 10.3389/fmars.2022.782680
Lin W., Zheng R., Liu B., Chen S., Lin M., Serres A., et al. (2023). Ranging pattern development of a declining delphinid population: A potential cascade effect of vessel activities. J. Environ. Manage. 330, 117120. doi: 10.1016/j.jenvman.2022.117120
Lu Y., Liu G., Cheung W. W. L., Xian Y., Chen W., Yu D. (2023). Anthropogenic footprints are invading global habitats of Indo-Pacific humpback dolphins. Geogr. Sustainability 4, 58–69. doi: 10.1016/j.geosus.2022.12.001
Lu F. H., Ni H. G., Liu F., Zeng E. Y. (2009). Occurrence of nutrients in riverine runoff of the Pearl River Delta, South China. J. Hydrology 376, 107–115. doi: 10.1016/j.jhydrol.2009.07.018
Lusseau D. (2005). Residency pattern of bottlenose dolphins Tursiops spp. in Milford Sound, New Zealand, is related to boat traffic. Mar. Ecol. Prog. Ser. 295, 265–272. doi: 10.3354/meps295265
Lusseau D. (2006). The shore-term behavioral reactions of bottlenose dolphins to interactions with boats in Doubtful Sound, New Zealand. Mar. Mammal Sci. 22, 802–818. doi: 10.1111/j.1748-7692.2006.00052.x
Ma L., Zhang J. (2009). Infrastructure development in a fast-growing economy: The People's Republic of China (Cheltenham, UK: Edward Elgar Publishing Limited). 264pp.
Manlik O., McDonald J. A., Mann J., Raudino H. C., Bejder L., Krützen M., et al. (2016). The relative importance of reproduction and survival for the conservation of two dolphin populations. Ecol. Evol. 6, 3496–3512. doi: 10.1002/ece3.2130
Marneweck D. G., Druce D. J., Cromsigt J. P. G. M., le Roux E., Somers M. J. (2022). The relative role of intrinsic and extrinsic drivers in regulating population change and survival of African wild dogs (Lycaon pictus). Mamm. Biol. 102, 1215–1229. doi: 10.1007/s42991-022-00281-z
Marsh H., Kasuya T. (1986). Evidence for reproductive senescence in female cetaceans. Rep. Int. Whaling Commission 8, 57–74.
Miller P. S. (2016). A population viability analysis for the Chinese white dolphin (Sousa chinensis) in the Pearl River Estuary: final report (Apple Valley MN: IUCN/SSC Conservation Breeding Specialist Group).
Murphy S., Law R. J., Deaville R., Barnett J., Perkins M. W., Brownlow A., et al. (2018). “Organochlorine contaminants and reproductive implication in cetaceans: A case study of the common dolphin,” in Marine Mammal Ecotoxicology. Eds. Fossi M. C., Panti C. (London and San Diego: Academic Press), 3–38. doi: 10.1016/C2016-0-03201-1
Oosthuizen W. C., Postma M., Altwegg R., Nevoux M., Pradel R., Bester M. N., et al. (2019b). Individual heterogeneity in life-history trade-offs with age at first reproduction in capital breeding elephant seals. Population Ecol. 61, 421–435. doi: 10.1002/1438-390X.12015
Oosthuizen W. C., Pradel R., Bester M. N., de Bruyn P. J. N. (2019a). Making use of multiple surveys: Estimating breeding probability using a multievent-robust design capture-recapture model. Ecol. Evol. 9, 836–848. doi: 10.1002/ece3.4828
Or C. (2016). Socio-spatial ecology of Indo-Pacific humpback dolphins (Sousa chinensis) in Hong Kong and Pearl River Estuary (Hong Kong: PhD dissertation, The University of Hong Kong).
Ouyang T., Kuang Y., Hu Z., Bo S. (2005). Urbanization in the pearl river delta economic zone, China. Int. J. Sustain. Dev. World Ecol. 12, 48–54. doi: 10.1080/13504500509469617
Pace R. M. III., Corkeron P. J., Kraus S. D. (2017). State–space mark–recapture estimates reveal a recent decline in abundance of North Atlantic right whales. Ecol. Evol. 7, 8730–8741. doi: 10.1002/ece3.3406
Parra G. J., Schick R., Corkeron P. J. (2006). Spatial distribution and environmental correlates of Australian snubfin and Indo-Pacific humpback dolphins. Ecography 29, 396–406. doi: 10.1111/j.2006.0906-7590.04411.x
Peel D., Bravington M., Kelly N., Double M. C. (2016). Designing an effective mark–recapture study of Antarctic blue whales. Ecol. Appl. 25, 1003–1015. doi: 10.1890/14-1169.1
Pine M. K., Wang D., Porter L., Wang K. (2018). Investigating the spatiotemporal variation of fish choruses to help identify important foraging habitat for Indo-Pacific humpback dolphins, Sousa chinensis. ICES J. Mar. Sci. 75, 510–518. doi: 10.1093/icesjms/fsx197
Pine M. K., Wang K., Wang D. (2017). Fine-scale habitat use in Indo-Pacific humpback dolphins, Sousa chinensis, may be more influenced by fish rather than vessels in the Pearl River Estuary, China. Mar. Mammal Sci. 33, 291–312. doi: 10.1111/mms.12366
Pine M. K., Wilson L., Jeffs A. G., McWhinnie L., Juanes F., Scuderi A., et al. (2021). A gulf in lockdown: How an enforced ban on recreational vessels increased dolphin and fish communication ranges. Global Change Biol. 27, 4839–4848. doi: 10.1111/gcb.15798
Rako N., Fortuna C. M., Holcer D., Mackelworth P., Nimak-Wood M., Pleslić G., et al. (2013). Leisure boating noise as a trigger for the displacement of the bottlenose dolphins of the Cres–Lošinj archipelago (northern Adriatic Sea, Croatia). Mar. pollut. Bull. 68, 77–84. doi: 10.1016/j.marpolbul.2012.12.019
Reeves R. R., Dalebout M. L., Jefferson T. A., Karczmarski L., Laidre K., O'Corry-Crowe G., et al. (2008). “Sousa chinensis,” in IUCN Red List of Threatened Species. Version 2013.2. <www.iucnredlist.org>. Downloaded on 18 April 2014.
Reid J. M., Bignal E. M., Bignal S., McCracken D. I., Bogdanova M. I., Monaghan P. (2008). Investigating patterns and processes of demographic variation: environmental correlates of pre-breeding survival in red-billed choughs Pyrrhocorax pyrrhocorax. J. Anim. Ecol. 77, 777–788. doi: 10.1111/j.1365-2656.2008.01400.x
Ritterson J. D., King D. I., Chandler R. B. (2021). Habitat-specific survival of golden-winged warblers Vermivora chrysoptera during the non-breeding season in an agricultural landscape. J. Avian Biol. 52, jav.02442. doi: 10.1111/jav.02442
Rotella J. J., Link W. A., Chambert T., Stauffer G. E., Garrott R. A. (2012). Evaluating the demographic buffering hypothesis with vital rates estimated for Weddell seals from 30 years of mark–recapture data. J. Anim. Ecol. 81, 162–173. doi: 10.1111/j.1365-2656.2011.01902.x
Sandercock B. K. (2006). Estimation of demographic parameters from live-encounter data: a summary review. J. Wildlife Manage. 70, 1504–1520. doi: 10.2193/0022-541X(2006)70[1504:EODPFL]2.0.CO;2
Schleimer A., Ramp C., Delarue J., Carpentier A., Bérubé M., Palsbøll P. J., et al. (2019). Decline in abundance and apparent survival rates of fin whales (Balaenoptera physalus) in the northern Gulf of St. Lawrence. Ecol. Evol. 9, 4231–4244. doi: 10.1002/ece3.5055
Schwartz C. J., Arnason A. N. (1996). A general methodology for the analysis of capture-recapture experiments in open populaions. Biometrics 52, 860–873. doi: 10.2307/2533048
Scrucca L., Fop M., Murphy T. B., Raftery A. E. (2016). mclust 5: Clustering, classification and density estimation using Gaussian finite mixture models. R J. 8, 289–317. doi: 10.32614/RJ-2016-021
Seber G. (1965). A note on the multiple±recapture census. Biometrika 52, 249–259. doi: 10.1093/biomet/52.1-2.249
Storch I. (1997). "The importance of scale in habitat conservation for an endangered species: The Capercaillie in central Europe" In Wildlife and Landscape Ecology Ed. Bissonette J. A. (New York, NY: Springer), 310–330. doi: 10.1007/978-1-4612-1918-7_12
Szott E. A., Brightwell K., Gibson Q. (2022). Assessment of social mixing and spatial overlap as a pathway for disease transmission in a northeast Florida estuarine dolphin community. Mamm. Biol. 102, 1267–1283. doi: 10.1007/s42991-022-00282-y
Urian K., Gorgone A., Read A., Balmer B., Wells R. S., Berggren P., et al. (2015). Recommendations for photo-identification methods used in capture-recapture models with cetaceans. Mar. Mammal Sci. 31, 298–321. doi: 10.1111/mms.12141
Verborgh P., Janssen E. H., Esteban R., Gauffier P., Freitasm L. (2022). Proposing a framework for monitoring demographic parameters in local cetacean populations: the case of short-finned pilot whales in Madeira. Mamm. Biol. 102, 1425–1444. doi: 10.1007/s42991-022-00266-y
Wang J. Y., Yang S. C., Fruet P. F., Daura-Jorge F. G., Secchi E. R. (2012). Mark-recapture analysis of the critically endangered eastern Taiwan Strait population of Indo-Pacific humpback dolphins (Sousa chinensis): implications for conservation. Bull. Mar. Sci. 88, 885–902. doi: 10.5343/bms.2010.1097
Wang Y., Hu J., Pan H., Li S., Failler P. (2016). An integrated model for marine fishery management in the Pearl River Estuary: Linking socio-economic systems and ecosystems. Mar. Policy 64, 135–147. doi: 10.1016/j.marpol.2015.11.014
Wang D., Lin Z. (2006). Spatial and temporal variations of fish community structure in the Pearl River Estuary waters. South China Fisheries Sci. 2, 37–45.
Wang W.-X., Rainbow P. S. (2020). Environmental pollution of the Pearl River Estuary, China (Berlin: Springer Nature). doi: 10.1007/978-3-662-61834-9
Wells R. S., Tornero V., Borrell A., Aguilar A., Rowles T. K., Rhinehart H. L., et al. (2005). Integrating life-history and reproductive success data to examine potential relationships with organochlorine compounds for bottlenose dolphins (Tursiops truncatus) in Sarasota Bay, Florida. Sci. Total Environ. 349, 106–119. doi: 10.1016/j.scitotenv.2005.01.010
Weng Q. (2007). A historical perspective of river basin management in the Pearl River Delta of China. J. Environ. Manage. 85, 1048–1062. doi: 10.1016/j.jenvman.2006.11.008
White G., Burnham K. (1999). Program MARK: survival estimation from populations of marked animals. Bird Study 46, S120–S139. doi: 10.1080/00063659909477239
Wilson B., Porter L., Gordon J., Hammond J., Hodgins N., Li W., et al. (2008). A Decade of Management Plans, Conservation initiatives and protective legislation for Chinese white dolphin (Sousa chinensis): An assessment of progress and recommendations for future management strategies in the Pearl River Estuary, China (Hong Kong: WWF Hong Kong). Workshop Report 7-11 April65pp.
World Bank (2015). East Asia's Changing Urban Landscape: Measuring a decade of spatial growth (Washington, DC: World Bank). Available at: http://www.worldbank.org/content/dam/Worldbank/Publications/Urban%20Development/EAP_Urban_Expansion_full_report_web.pdf. 157 pp.
Wu C. S., Yang S., Huang S., Mu J. (2016). Delta changes in the Pearl River estuary and its response to human activities, (1954–2008). Quaternary Int. 392, 147–154. doi: 10.1016/j.quaint.2015.04.009
Yu J., Chen Z., Xu S. (2016). Land reclamation and its impact on fisheries resrouces in the Nansha wetland of Pearl River Estuary. J. Fishery Sci. China 23, 661–671. doi: 10.3724/SP.J.1118.2016.15352
Zeng Q., Lin W., Dai Y., Zhong M., Wang X., Zhu Q. (2020). Modeling demographic parameters of an edge-of-range population of Indo-Pacific humpback dolphin in Xiamen Bay, China. Regional Stud. Mar. Sci. 40, 101462. doi: 10.1016/j.rsma.2020.101462
Zhang X., Huang H., Wang P., Chen M., Wang J., Sun Q. (2016). Change analysis of coastline and sea reclamation in Pearl River Estuary from 1973-2015. Trans. Oceanology Limnology 5, 9–15.
Zhang X., Yu R.-Q., Lin W., Gui D., Sun X., Yu X., et al. (2019). Stable isotope analyses reveal anthropogenically driven spatial and trophic changes to Indo-Pacific humpback dolphins in the Pearl River Estuary, China. Sci. Total Environ. 651, 1029–1037. doi: 10.1016/j.scitotenv.2018.09.256
Zhou H., Peng X., Pan J. (2004). Distribution, source and enrichment of some chemical elements in sediments of the Pearl River Estuary, China. Continental Shelf Res. 24, 1857–1875. doi: 10.1016/j.csr.2004.06.012
Zhou L., Wang G., Kuang T., Guo D., Li G. (2019). Fish assemblage in the Pearl River Estuary: Spatial-seasonal variation, environmental influence and trends over the past three decades. J. Appl. Ichthyology 35, 884–895. doi: 10.1111/jai.13912
Zhu J., Wang G., Zhang J., Huang T. (2013). Remote sensing investigation and recent evolution analysis of Pearl River delta coastline. Remote Sens. Land Resour. 25, 130–137. doi: 10.6046/gtzyyg.2013.03.22
Keywords: Sousa chinensis, inshore cetaceans, Lingding Bay/Pearl River Estuary, mark-recapture population modeling, population size estimate, apparent survival rates, spatial heterogeneity, declining abundance
Citation: Lin W, Karczmarski L, Chan SCY, Zheng R, Ho Y-W and Mo Y (2024) Population parameters and heterogeneity in survival rates of Indo-Pacific humpback dolphins in a heavily urbanized coastal region of southeast China: implications for conservation. Front. Mar. Sci. 11:1252661. doi: 10.3389/fmars.2024.1252661
Received: 04 July 2023; Accepted: 22 January 2024;
Published: 20 March 2024.
Edited by:
Mark Meekan, University of Western Australia, AustraliaReviewed by:
Thomas A. Jefferson, Clymene Enterprises, United StatesPavel Gol’din, National Academy of Sciences of Ukraine (NAN Ukraine), Ukraine
Copyright © 2024 Lin, Karczmarski, Chan, Zheng, Ho and Mo. This is an open-access article distributed under the terms of the Creative Commons Attribution License (CC BY). The use, distribution or reproduction in other forums is permitted, provided the original author(s) and the copyright owner(s) are credited and that the original publication in this journal is cited, in accordance with accepted academic practice. No use, distribution or reproduction is permitted which does not comply with these terms.
*Correspondence: Wenzhi Lin, linwz@idsse.ac.cn; Leszek Karczmarski, leszek@cetacea-institute.org
†These authors have contributed equally to this work
‡ORCID: Wenzhi Lin, orcid.org/0000-0002-0477-0753
Leszek Karczmarski, orcid.org/0000-0001-9712-1172
Stephen C.Y. Chan, orcid.org/0000-0002-0157-9574